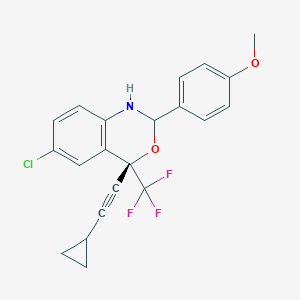
Efavirenz impurity I
Overview
Description
Efavirenz Impurity I, chemically identified as (2S)-(2-Amino-5-chlorophenyl)-4-cyclopropyl-1,1,1-trifluoro-3-butyn-2-ol (AMCOL), is a critical process-related and degradation impurity in the synthesis of efavirenz, a non-nucleoside reverse transcriptase inhibitor (NNRTI) used in HIV treatment . AMCOL arises during the cyclopropanation step of efavirenz synthesis (Figure 1a) and can also form via degradation under specific conditions (Figure 1b) . Its aminoaryl functional group is a structural alert for genotoxicity, leading to its classification as a Potential Genotoxic Impurity (PGI) requiring strict control per International Council for Harmonisation (ICH) guidelines . Regulatory agencies mandate its trace-level quantification (<1.5 µg/day) to ensure patient safety .
Analytical methods for AMCOL determination, such as LC-MS/MS, have been developed to achieve the required sensitivity (limit of detection: 0.015 ppm) . In contrast, traditional HPLC methods used for other efavirenz impurities lack the specificity and sensitivity for AMCOL quantification at these levels .
Preparation Methods
Synthetic Routes and Reaction Mechanisms
The synthesis of Efavirenz Impurity I primarily involves cyclization or condensation reactions under controlled conditions.
Acid-Catalyzed Cyclization
A patented method (CN107474014A) describes the preparation of the quinoline variant (Formula III) via copper-catalyzed cyclization of Formula II in tetrahydrofuran (THF) or toluene. The reaction proceeds at 60°C for 2–4 hours, yielding 81–86% of the impurity . The mechanism involves protonation of the amino group, followed by intramolecular nucleophilic attack and dehydration (Figure 1).
Table 1: Representative Cyclization Conditions
Catalyst | Solvent | Temperature (°C) | Time (h) | Yield (%) |
---|---|---|---|---|
Copper acetate | THF | 60 | 2 | 85.6 |
Trifluoroacetic acid copper | Toluene | 60 | 3 | 82.6 |
Iron chloride | Acetonitrile | 60 | 4 | 81.8 |
Carbamate Formation
An alternative route involves the reaction of efavirenz intermediates with chlorobutyl carbamates in tetrahydrofuran, facilitated by microwave irradiation. This method isolates the carbamate impurity (4-Chlorobutyl)[4-Chloro-2(4-Cyclopropyl-1,1,1-Trifluoro-but-3-yn-2-ol)Phenyl] carbamate with >95% purity via preparative HPLC .
Catalytic Systems and Solvent Selection
Catalysts and solvents critically influence reaction efficiency and impurity profile.
Catalyst Optimization
Copper-based catalysts (e.g., copper acetate, trifluoroacetic acid copper) demonstrate superior efficacy in cyclization reactions compared to iron or zinc salts. For instance, copper acetate in THF achieves 85.6% yield, whereas iron chloride in acetonitrile yields only 81.8% . Trifluoroacetic acid serves dual roles as a catalyst and proton donor, enhancing reaction kinetics .
Solvent Effects
Polar aprotic solvents (THF, acetonitrile) favor cyclization due to improved solubility of intermediates, while non-polar solvents (toluene) reduce byproduct formation. Post-processing in n-heptane at 0°C ensures high-purity crystallization (>98% by HPLC) .
Post-Processing and Crystallization Techniques
Isolation of this compound requires meticulous post-processing:
-
Cooling and Extraction : Reaction mixtures are cooled to 25°C, diluted with water, and extracted with ethyl acetate .
-
Washing and Concentration : Organic phases are washed with brine and concentrated under reduced pressure.
-
Crystallization : Crude product is dissolved in n-heptane and cooled to 0°C, yielding crystalline impurity .
Table 2: Crystallization Parameters
Solvent | Cooling Temperature (°C) | Purity (%) | Yield (%) |
---|---|---|---|
n-Heptane | 0 | 98.5 | 82.1 |
Hexane | -10 | 97.8 | 80.3 |
Cyclohexane | 5 | 96.2 | 78.9 |
Analytical Characterization and Validation
Advanced analytical techniques ensure accurate identification and quantification of this compound.
Chromatographic Methods
A validated LC-MS/MS method employs a C18 column (150 × 4.6 mm, 3.5 µm) with a gradient elution of 0.1% formic acid and acetonitrile. Impurity I elutes at 22.0 min (MRM transition: 364.15→291.25), achieving a limit of detection (LOD) of 0.05 ppm .
Table 3: Validation Parameters for LC-MS/MS
Parameter | Impurity I | Acceptance Criteria |
---|---|---|
Linearity (R²) | 0.999 | ≥0.990 |
LOD (ppm) | 0.05 | ≤0.10 |
LOQ (ppm) | 0.15 | ≤0.30 |
Precision (% RSD) | 2.6 | ≤5.0 |
Spectroscopic Characterization
-
NMR : ¹H NMR (400 MHz, CDCl₃) δ 7.45 (d, J=8.4 Hz, 1H), 6.95 (s, 1H), 5.20 (s, 1H) .
-
MS : m/z 364.15 [M+H]⁺, consistent with the molecular formula C₁₄H₁₁ClF₃NO .
Comparative Analysis of Methodologies
Cyclization methods using copper catalysts in THF offer higher yields (85.6%) and scalability compared to carbamate routes (75–80%) . However, the latter provides superior selectivity for structural analogs.
Challenges and Optimization Strategies
Key challenges include low yields in polar solvents (e.g., acetonitrile) and byproduct formation during crystallization. Optimization strategies involve:
-
Catalyst Screening : Testing palladium or nickel catalysts for enhanced efficiency.
-
Green Solvents : Replacing THF with cyclopentyl methyl ether (CPME) to reduce toxicity .
Regulatory Considerations and Quality Control
Current USP and ICH guidelines mandate impurity levels <0.15%, necessitating rigorous in-process monitoring via HPLC or LC-MS/MS . Accelerated stability studies (40°C/75% RH) confirm impurity I’s stability under storage conditions .
Chemical Reactions Analysis
Types of Reactions
Efavirenz impurity I can undergo various chemical reactions, including:
Oxidation: This reaction involves the addition of oxygen or the removal of hydrogen, often using oxidizing agents such as hydrogen peroxide or potassium permanganate.
Reduction: This reaction involves the addition of hydrogen or the removal of oxygen, typically using reducing agents like sodium borohydride or lithium aluminum hydride.
Common Reagents and Conditions
Common reagents used in these reactions include acids, bases, solvents like methanol and acetonitrile, and catalysts such as palladium on carbon. The conditions vary depending on the desired reaction, with temperature ranges from room temperature to elevated temperatures and pressures .
Major Products Formed
The major products formed from these reactions depend on the specific reaction pathway. For example, oxidation of this compound may lead to the formation of hydroxylated or carboxylated derivatives, while reduction may yield dehalogenated products .
Scientific Research Applications
Efavirenz impurity I has several scientific research applications, including:
Mechanism of Action
its presence can influence the overall pharmacological profile of Efavirenz by potentially interacting with the same molecular targets, such as the reverse transcriptase enzyme in HIV-1 . The pathways involved may include binding to the enzyme’s active site and inhibiting its function, similar to Efavirenz .
Comparison with Similar Compounds
Efavirenz Impurity I is structurally and functionally distinct from other efavirenz-related impurities and genotoxic compounds. Below is a detailed comparison:
Structural and Functional Comparison
Key Differences:
Genotoxicity Mechanism: AMCOL’s aminoaryl group directly interacts with DNA, triggering genotoxicity . SR695 and Methyl Impurity lack structural alerts for genotoxicity, resulting in lower regulatory scrutiny . Phenylhydrazine, like AMCOL, is genotoxic but requires distinct analytical methods (e.g., LC-ICP-MS) due to its inorganic nature .
Analytical Challenges :
- AMCOL necessitates LC-MS/MS for trace-level detection, whereas SR695 and Methyl Impurity are quantified via conventional HPLC .
- Supercritical fluid chromatography (SFC) has been explored for efavirenz impurity profiling but lacks validation for AMCOL .
Regulatory Limits: AMCOL’s threshold (0.15%) aligns with ICH’s Threshold of Toxicological Concern (TTC) for genotoxins, whereas non-genotoxic impurities like SR695 have higher limits .
Pharmacokinetic and Stability Considerations
- Degradation Pathways: AMCOL forms via hydrolysis of efavirenz’s oxazinanone ring, a process accelerated under acidic or oxidative conditions . In contrast, SR695 and Methyl Impurity arise from incomplete synthesis steps .
Research Findings and Regulatory Implications
Methodology Advancements : LC-MS/MS methods for AMCOL achieve a linear range of 0.015–1.5 ppm, outperforming pharmacopeial HPLC methods .
Comparative Stability : AMCOL is more stable in solid-state formulations than in solution, unlike Methyl Impurity, which degrades under UV light .
Biological Activity
Efavirenz, a non-nucleoside reverse transcriptase inhibitor (NNRTI), is widely used in the treatment of HIV. However, the presence of impurities, particularly Efavirenz Impurity I (also known as AMCOL), raises concerns regarding its biological activity and potential genotoxic effects. This article explores the biological activity associated with this compound, including its genotoxicity, analytical methods for detection, and implications for patient safety.
Overview of this compound
This compound is identified as a degradation impurity in the synthesis of efavirenz. It is characterized as a potential genotoxic impurity (PGI) due to its aminoaryl derivative structure, which is associated with genotoxic activity. Regulatory authorities have emphasized the need to monitor and control such impurities in pharmaceutical formulations to ensure patient safety.
Mechanisms of Genotoxicity
The genotoxic effects of this compound are primarily attributed to its ability to induce DNA damage. Studies have indicated that compounds with aminoaryl structures can interact with cellular DNA, leading to mutations and potential carcinogenic outcomes. The threshold of toxicological concern (TTC) for this impurity has been set at 1.5 µg/day based on its risk profile .
Analytical Methods for Detection
To assess the levels of this compound in pharmaceutical products, sensitive analytical techniques have been developed:
- Liquid Chromatography-Tandem Mass Spectrometry (LC–MS/MS) : This method allows for the trace-level quantification of AMCOL, providing excellent sensitivity and specificity. The method has been validated according to International Council on Harmonisation (ICH) guidelines, ensuring robust performance in detecting impurity levels below regulatory thresholds .
- Reverse Phase High-Performance Liquid Chromatography (RP-HPLC) : This technique has also been employed to quantify genotoxic N-nitrosamines in efavirenz formulations. The results demonstrated satisfactory linearity and accuracy, confirming the presence of these impurities at low concentrations .
Clinical Implications
Research has shown that patients receiving efavirenz-based antiretroviral therapy may experience varying metabolic responses compared to those on alternative therapies like dolutegravir. A study highlighted that efavirenz treatment was associated with altered metabolite levels and inflammatory markers, which could be influenced by the presence of impurities such as this compound .
Comparative Studies
A comparative analysis between efavirenz and its impurities revealed significant differences in biological activity. Specifically, metabolites associated with efavirenz treatment exhibited altered concentrations in patients, suggesting that impurities might contribute to the overall pharmacological profile and side effects experienced by patients .
Data Summary
The following table summarizes key findings related to the biological activity and detection methods for this compound:
Parameter | Details |
---|---|
Chemical Structure | Aminoaryl derivative |
Genotoxicity | Induces DNA damage |
TTC Limit | 1.5 µg/day |
Detection Method | LC–MS/MS |
Validation Standards | ICH guidelines |
Clinical Observations | Altered metabolite levels in patients |
Q & A
Basic Questions
Q. What analytical methods are recommended for identifying and quantifying Efavirenz Impurity I in drug formulations?
To detect and quantify this compound, reverse-phase HPLC coupled with UV or DAD detection is widely employed. Critical parameters include column selection (e.g., C18 or phenyl-hexyl stationary phases) and mobile phase optimization (e.g., acetonitrile-phosphate buffer systems) to resolve impurities like SR695 and trans-alkene derivatives . For example, Weissburg et al. (2002) achieved baseline separation of SR695 using a gradient elution protocol with a phenyl column . The Brazilian Pharmacopoeia specifies a quantification factor (1.1×) for trans-alkene impurity, limiting it to ≤0.15% .
Q. What synthetic pathways or degradation mechanisms lead to the formation of this compound?
Impurity I typically arises during synthesis or storage via cyclization, oxidation, or isomerization. For instance, cyclization intermediates formed during catalytic steps (e.g., enzyme-mediated reactions) can generate benzoxazine derivatives, a common impurity class . Stability studies under forced degradation (acid/base hydrolysis, thermal stress) reveal degradation pathways, with HPLC-MS used to characterize byproducts .
Q. How do pharmacopeial standards regulate this compound limits?
The Brazilian Pharmacopoeia (6th edition) sets a threshold of ≤0.15% for trans-alkene impurity (likely Impurity I) and ≤0.5% for total unspecified impurities. Compliance requires method validation per ICH Q2(R1), including specificity, linearity, and precision .
Advanced Research Questions
Q. What methodological challenges arise in developing stability-indicating assays for this compound?
Key challenges include resolving co-eluting impurities and ensuring method robustness. Montgomery et al. (2001) highlighted the impact of pH and organic modifier concentration on peak symmetry and resolution . Advanced approaches like Design of Experiments (DoE) optimize chromatographic conditions, while LC-MS/MS enhances sensitivity for trace impurities (e.g., genotoxic compounds at ppm levels) .
Q. How can researchers adapt HPLC methods for impurity profiling in biological matrices (e.g., plasma)?
Biological samples require protein precipitation or solid-phase extraction to reduce matrix interference. Mogatle & Kanfer (2009) validated a plasma method using acetonitrile precipitation and sodium dihydrogen orthophosphate buffer, achieving a lower quantification limit (LOQ) of 50 ng/mL for Efavirenz and its impurities . Method transfer to LC-MS/MS further improves specificity for low-abundance impurities .
Q. What strategies resolve contradictory data on Efavirenz-associated neuropsychiatric effects and impurity contributions?
Contradictions in clinical data (e.g., efavirenz-linked suicidal ideation vs. no association in cohort studies) require systematic reviews and meta-analyses to assess confounding variables (e.g., CYP2B6 polymorphisms). Pharmacokinetic studies by Csajka et al. (2003) linked high plasma Efavirenz levels to toxicity, suggesting impurities may exacerbate exposure variability . In vitro neurotoxicity assays (e.g., neuronal cell models) could isolate impurity-specific effects .
Q. How do CYP polymorphisms influence the pharmacokinetics of Efavirenz and its impurities?
CYP2B6 516G→T and CYP2A6 variants reduce Efavirenz metabolism, increasing plasma concentrations and potential impurity accumulation. Genotyping studies (e.g., Schetz et al., 2012) recommend personalized dosing to mitigate toxicity risks . In vitro hepatocyte models with CYP isoform inhibitors clarify metabolic pathways for impurities .
Q. What validation parameters are critical for quantifying genotoxic impurities like this compound?
ICH M7 guidelines mandate validation of specificity, sensitivity (LOD/LOQ ≤ 30 ppm), and linearity (R² ≥ 0.99). Jaishetty et al. (2015) validated an LC-MS/MS method for a genotoxic impurity in Efavirenz, achieving an LOQ of 0.001% w/w using a deuterated internal standard . Forced degradation studies confirm method stability-indicating capability .
Q. How do impurities impact Efavirenz’s long-term stability in formulation studies?
Accelerated stability testing (40°C/75% RH) monitors impurity growth over time. Montgomery et al. (2001) reported that oxidation impurities increase under light exposure, necessitating protective packaging . Degradation kinetics models (e.g., Arrhenius equation) predict shelf-life and impurity thresholds under varying storage conditions .
Q. What preclinical models assess the biological activity or toxicity of this compound?
In vitro cytotoxicity assays (e.g., MTT in HepG2 cells) and in vivo rodent models evaluate impurity-specific effects. Schetz et al. (2012) used dopamine receptor binding assays to link Efavirenz’s psychoactivity to impurity interactions with CNS targets . Genotoxicity assays (Ames test, micronucleus) are critical for ICH compliance .
Q. Methodological Recommendations
- Chromatography : Optimize mobile phase pH (2.5–3.5) and column temperature (25–40°C) to enhance impurity resolution .
- Mass Spectrometry : Use MRM mode for trace analysis, with ESI+ ionization for Efavirenz derivatives .
- Data Analysis : Apply multivariate statistics (e.g., PCA) to correlate impurity profiles with clinical outcomes .
Properties
IUPAC Name |
(4S)-6-chloro-4-(2-cyclopropylethynyl)-2-(4-methoxyphenyl)-4-(trifluoromethyl)-1,2-dihydro-3,1-benzoxazine | |
---|---|---|
Source | PubChem | |
URL | https://pubchem.ncbi.nlm.nih.gov | |
Description | Data deposited in or computed by PubChem | |
InChI |
InChI=1S/C21H17ClF3NO2/c1-27-16-7-4-14(5-8-16)19-26-18-9-6-15(22)12-17(18)20(28-19,21(23,24)25)11-10-13-2-3-13/h4-9,12-13,19,26H,2-3H2,1H3/t19?,20-/m0/s1 | |
Source | PubChem | |
URL | https://pubchem.ncbi.nlm.nih.gov | |
Description | Data deposited in or computed by PubChem | |
InChI Key |
UTEUZELNJWOZOI-ANYOKISRSA-N | |
Source | PubChem | |
URL | https://pubchem.ncbi.nlm.nih.gov | |
Description | Data deposited in or computed by PubChem | |
Canonical SMILES |
COC1=CC=C(C=C1)C2NC3=C(C=C(C=C3)Cl)C(O2)(C#CC4CC4)C(F)(F)F | |
Source | PubChem | |
URL | https://pubchem.ncbi.nlm.nih.gov | |
Description | Data deposited in or computed by PubChem | |
Isomeric SMILES |
COC1=CC=C(C=C1)C2NC3=C(C=C(C=C3)Cl)[C@@](O2)(C#CC4CC4)C(F)(F)F | |
Source | PubChem | |
URL | https://pubchem.ncbi.nlm.nih.gov | |
Description | Data deposited in or computed by PubChem | |
Molecular Formula |
C21H17ClF3NO2 | |
Source | PubChem | |
URL | https://pubchem.ncbi.nlm.nih.gov | |
Description | Data deposited in or computed by PubChem | |
Molecular Weight |
407.8 g/mol | |
Source | PubChem | |
URL | https://pubchem.ncbi.nlm.nih.gov | |
Description | Data deposited in or computed by PubChem | |
CAS No. |
209414-26-6 | |
Record name | SG-275 | |
Source | ChemIDplus | |
URL | https://pubchem.ncbi.nlm.nih.gov/substance/?source=chemidplus&sourceid=0209414266 | |
Description | ChemIDplus is a free, web search system that provides access to the structure and nomenclature authority files used for the identification of chemical substances cited in National Library of Medicine (NLM) databases, including the TOXNET system. | |
Record name | SG-275 | |
Source | FDA Global Substance Registration System (GSRS) | |
URL | https://gsrs.ncats.nih.gov/ginas/app/beta/substances/S40TCL042S | |
Description | The FDA Global Substance Registration System (GSRS) enables the efficient and accurate exchange of information on what substances are in regulated products. Instead of relying on names, which vary across regulatory domains, countries, and regions, the GSRS knowledge base makes it possible for substances to be defined by standardized, scientific descriptions. | |
Explanation | Unless otherwise noted, the contents of the FDA website (www.fda.gov), both text and graphics, are not copyrighted. They are in the public domain and may be republished, reprinted and otherwise used freely by anyone without the need to obtain permission from FDA. Credit to the U.S. Food and Drug Administration as the source is appreciated but not required. | |
Disclaimer and Information on In-Vitro Research Products
Please be aware that all articles and product information presented on BenchChem are intended solely for informational purposes. The products available for purchase on BenchChem are specifically designed for in-vitro studies, which are conducted outside of living organisms. In-vitro studies, derived from the Latin term "in glass," involve experiments performed in controlled laboratory settings using cells or tissues. It is important to note that these products are not categorized as medicines or drugs, and they have not received approval from the FDA for the prevention, treatment, or cure of any medical condition, ailment, or disease. We must emphasize that any form of bodily introduction of these products into humans or animals is strictly prohibited by law. It is essential to adhere to these guidelines to ensure compliance with legal and ethical standards in research and experimentation.