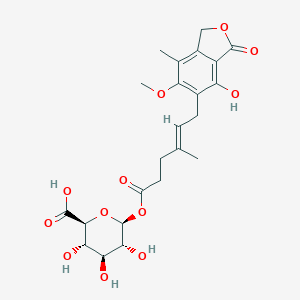
Mycophenolic acid acyl glucuronide
Overview
Description
Mycophenolic acid acyl glucuronide is a metabolite of mycophenolic acid, which is the active moiety of the prodrug mycophenolate mofetil. Mycophenolic acid is widely used in immunosuppressive regimens, particularly after kidney, liver, or heart transplantation. Mycophenolic acid is metabolized predominantly to the inactive 7-O-glucuronide, but a minor fraction is converted to the pharmacologically active acyl glucuronide .
Mechanism of Action
Target of Action
Mycophenolic acid acyl glucuronide (AcMPAG) is a metabolite of Mycophenolic acid (MPA), a potent immunosuppressant agent . The primary target of MPA and its metabolites is the enzyme inosine monophosphate dehydrogenase (IMPDH) . IMPDH plays a crucial role in the de novo pathway of guanosine nucleotide biosynthesis, which is essential for the proliferation of T- and B-lymphocytes .
Mode of Action
MPA, and by extension AcMPAG, acts as a selective, noncompetitive, and reversible inhibitor of IMPDH . It blocks the conversion of inosine-5-phosphate and xanthine-5-phosphate to guanosine-5-phosphate . By inhibiting IMPDH, MPA interferes with the de novo pathway of guanosine nucleotide biosynthesis, leading to a decrease in the proliferation of T- and B-lymphocytes .
Biochemical Pathways
The inhibition of IMPDH disrupts the de novo pathway of guanosine nucleotide biosynthesis, which is a critical pathway for DNA and RNA synthesis in lymphocytes . This disruption leads to a decrease in lymphocyte proliferation, thereby exerting an immunosuppressive effect .
Pharmacokinetics
MPA is metabolized primarily by glucuronidation in the liver to form MPA glucuronide (MPAG) and AcMPAG . These metabolites are primarily produced by UDP-glucuronosyltransferases UGT1A9 and 2B7 . The majority of MPA metabolites are eliminated via the urine, with partial elimination also occurring in the bile mediated by multidrug resistance-associated protein 2 (MRP2), followed by enterohepatic recirculation . The pharmacokinetics of MPA exhibit a large inter- and intra-individual variability .
Result of Action
The result of the action of MPA and its metabolites is a decrease in the proliferation of T- and B-lymphocytes . This leads to a reduction in the immune response, making MPA and its metabolites effective in preventing organ transplant rejections .
Action Environment
The action of MPA and its metabolites can be influenced by various environmental factors. For instance, decreased creatinine clearance can increase the MPAG concentration, followed by an increased MPA concentration, thereby decreasing IMPDH activity . Additionally, conditions like diarrhea can decrease the enterohepatic circulation of MPAG, consequently reducing MPA concentration . Therefore, dosage adjustment based on plasma MPA concentration may be required, especially for patients with renal dysfunction and/or diarrhea .
Biochemical Analysis
Biochemical Properties
Mycophenolic acid acyl glucuronide interacts with transport systems responsible for the excretion of organic anions in the basolateral membrane of the human kidney . It also interacts with renal organic anion transporters hOAT1 and hOAT3, potentially interfering with the renal secretion of antiviral drugs, cortisol, and other organic anions .
Cellular Effects
This compound has been found to possess pharmacologic and potentially proinflammatory activity in vitro . It has been suggested that it may interfere with the renal secretion of antiviral drugs, cortisol, and other organic anions .
Molecular Mechanism
This compound’s mechanism of action involves the selective inhibition of T-lymphocyte proliferation at the S-phase . It also potently interacts with renal organic anion transporters hOAT1 and hOAT3 .
Temporal Effects in Laboratory Settings
This compound was found to undergo hydrolysis when samples were stored up to 24 hours at room temperature or up to 30 days at 4°C or -20°C. Acidified samples (pH 2.5) were stable up to 30 days at -20°C .
Metabolic Pathways
This compound is primarily produced by UGT1A9 and 2B7 . It is a part of the glucuronidation pathway, a major metabolic pathway mediated by intestinal and liver UDP glucuronosyl transferases (UGTs) .
Transport and Distribution
This compound interacts with transport systems responsible for the excretion of organic anions in the basolateral membrane of the human kidney . It also interacts with renal organic anion transporters hOAT1 and hOAT3 .
Subcellular Localization
This compound esterase, which catalyzes the deglucuronidation of this compound, is localized in the mitochondrion .
Preparation Methods
Synthetic Routes and Reaction Conditions: The preparation of mycophenolic acid acyl glucuronide involves the conjugation of mycophenolic acid with glucuronic acid. This reaction is typically catalyzed by uridine diphosphate-glucuronosyltransferase enzymes. The reaction conditions often include the presence of a suitable buffer and co-factors necessary for enzyme activity .
Industrial Production Methods: Industrial production of this compound follows similar principles but on a larger scale. The process involves the use of bioreactors where mycophenolic acid is incubated with glucuronic acid and the necessary enzymes under controlled conditions to optimize yield and purity .
Chemical Reactions Analysis
Types of Reactions: Mycophenolic acid acyl glucuronide undergoes several types of chemical reactions, including hydrolysis and transacylation. These reactions are significant because they can lead to the formation of protein adducts, which are associated with the compound’s potential toxicity .
Common Reagents and Conditions:
Hydrolysis: This reaction occurs in the presence of water and can be catalyzed by enzymes or acidic conditions.
Transacylation: This reaction involves the transfer of the acyl group to another molecule, often facilitated by the presence of nucleophiles.
Major Products:
Hydrolysis: The major product is mycophenolic acid.
Transacylation: The products vary depending on the nucleophile involved but can include various acylated compounds.
Scientific Research Applications
Mycophenolic acid acyl glucuronide has several scientific research applications:
Comparison with Similar Compounds
Mycophenolic acid 7-O-glucuronide: This is the major metabolite of mycophenolic acid and is pharmacologically inactive.
Acyl glucosides: These compounds undergo similar degradation reactions and have comparable potential for toxicity.
Uniqueness: Mycophenolic acid acyl glucuronide is unique due to its pharmacological activity and its ability to form protein adducts, which distinguishes it from the more common and inactive 7-O-glucuronide metabolite .
Properties
IUPAC Name |
(2S,3S,4S,5R,6S)-3,4,5-trihydroxy-6-[(E)-6-(4-hydroxy-6-methoxy-7-methyl-3-oxo-1H-2-benzofuran-5-yl)-4-methylhex-4-enoyl]oxyoxane-2-carboxylic acid | |
---|---|---|
Source | PubChem | |
URL | https://pubchem.ncbi.nlm.nih.gov | |
Description | Data deposited in or computed by PubChem | |
InChI |
InChI=1S/C23H28O12/c1-9(4-6-11-15(25)14-12(8-33-22(14)31)10(2)19(11)32-3)5-7-13(24)34-23-18(28)16(26)17(27)20(35-23)21(29)30/h4,16-18,20,23,25-28H,5-8H2,1-3H3,(H,29,30)/b9-4+/t16-,17-,18+,20-,23+/m0/s1 | |
Source | PubChem | |
URL | https://pubchem.ncbi.nlm.nih.gov | |
Description | Data deposited in or computed by PubChem | |
InChI Key |
QBMSTEZXAMABFF-UEARNRKISA-N | |
Source | PubChem | |
URL | https://pubchem.ncbi.nlm.nih.gov | |
Description | Data deposited in or computed by PubChem | |
Canonical SMILES |
CC1=C2COC(=O)C2=C(C(=C1OC)CC=C(C)CCC(=O)OC3C(C(C(C(O3)C(=O)O)O)O)O)O | |
Source | PubChem | |
URL | https://pubchem.ncbi.nlm.nih.gov | |
Description | Data deposited in or computed by PubChem | |
Isomeric SMILES |
CC1=C2COC(=O)C2=C(C(=C1OC)C/C=C(\C)/CCC(=O)O[C@H]3[C@@H]([C@H]([C@@H]([C@H](O3)C(=O)O)O)O)O)O | |
Source | PubChem | |
URL | https://pubchem.ncbi.nlm.nih.gov | |
Description | Data deposited in or computed by PubChem | |
Molecular Formula |
C23H28O12 | |
Source | PubChem | |
URL | https://pubchem.ncbi.nlm.nih.gov | |
Description | Data deposited in or computed by PubChem | |
DSSTOX Substance ID |
DTXSID501343826 | |
Record name | Mycophenolic acid O-acyl-glucuronide | |
Source | EPA DSSTox | |
URL | https://comptox.epa.gov/dashboard/DTXSID501343826 | |
Description | DSSTox provides a high quality public chemistry resource for supporting improved predictive toxicology. | |
Molecular Weight |
496.5 g/mol | |
Source | PubChem | |
URL | https://pubchem.ncbi.nlm.nih.gov | |
Description | Data deposited in or computed by PubChem | |
CAS No. |
99043-04-6 | |
Record name | Mycophenolic acid acyl glucuronide | |
Source | ChemIDplus | |
URL | https://pubchem.ncbi.nlm.nih.gov/substance/?source=chemidplus&sourceid=0099043046 | |
Description | ChemIDplus is a free, web search system that provides access to the structure and nomenclature authority files used for the identification of chemical substances cited in National Library of Medicine (NLM) databases, including the TOXNET system. | |
Record name | MYCOPHENOLIC ACID ACYL GLUCURONIDE | |
Source | FDA Global Substance Registration System (GSRS) | |
URL | https://gsrs.ncats.nih.gov/ginas/app/beta/substances/B96732OXNA | |
Description | The FDA Global Substance Registration System (GSRS) enables the efficient and accurate exchange of information on what substances are in regulated products. Instead of relying on names, which vary across regulatory domains, countries, and regions, the GSRS knowledge base makes it possible for substances to be defined by standardized, scientific descriptions. | |
Explanation | Unless otherwise noted, the contents of the FDA website (www.fda.gov), both text and graphics, are not copyrighted. They are in the public domain and may be republished, reprinted and otherwise used freely by anyone without the need to obtain permission from FDA. Credit to the U.S. Food and Drug Administration as the source is appreciated but not required. | |
Retrosynthesis Analysis
AI-Powered Synthesis Planning: Our tool employs the Template_relevance Pistachio, Template_relevance Bkms_metabolic, Template_relevance Pistachio_ringbreaker, Template_relevance Reaxys, Template_relevance Reaxys_biocatalysis model, leveraging a vast database of chemical reactions to predict feasible synthetic routes.
One-Step Synthesis Focus: Specifically designed for one-step synthesis, it provides concise and direct routes for your target compounds, streamlining the synthesis process.
Accurate Predictions: Utilizing the extensive PISTACHIO, BKMS_METABOLIC, PISTACHIO_RINGBREAKER, REAXYS, REAXYS_BIOCATALYSIS database, our tool offers high-accuracy predictions, reflecting the latest in chemical research and data.
Strategy Settings
Precursor scoring | Relevance Heuristic |
---|---|
Min. plausibility | 0.01 |
Model | Template_relevance |
Template Set | Pistachio/Bkms_metabolic/Pistachio_ringbreaker/Reaxys/Reaxys_biocatalysis |
Top-N result to add to graph | 6 |
Feasible Synthetic Routes
Disclaimer and Information on In-Vitro Research Products
Please be aware that all articles and product information presented on BenchChem are intended solely for informational purposes. The products available for purchase on BenchChem are specifically designed for in-vitro studies, which are conducted outside of living organisms. In-vitro studies, derived from the Latin term "in glass," involve experiments performed in controlled laboratory settings using cells or tissues. It is important to note that these products are not categorized as medicines or drugs, and they have not received approval from the FDA for the prevention, treatment, or cure of any medical condition, ailment, or disease. We must emphasize that any form of bodily introduction of these products into humans or animals is strictly prohibited by law. It is essential to adhere to these guidelines to ensure compliance with legal and ethical standards in research and experimentation.