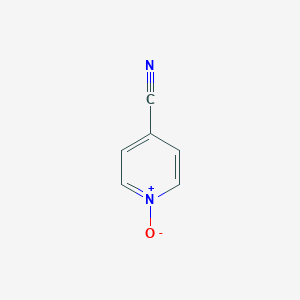
4-Cyanopyridine N-oxide
Overview
Description
4-Cyanopyridine N-oxide is an organic compound with the molecular formula C6H4N2O. It is a derivative of pyridine, where the nitrogen atom in the pyridine ring is oxidized, and a cyano group is attached to the fourth position of the ring. This compound is known for its utility in various chemical reactions and its role as a building block in organic synthesis .
Mechanism of Action
Target of Action
4-Cyanopyridine N-oxide, also known as Isonicotinonitrile 1-oxide, is a heterocyclic compound It’s known to be used as a ligand for other metals or compounds for the formation of new dimeric complexes .
Mode of Action
It’s known to act as a monodentate ligand in transition metal complexes via the pyridine nitrogen atom, or as a bidentate ligand via both nitrogen atoms, resulting in a linear bridge between two metal atoms . This suggests that the compound may interact with its targets to form complex structures, potentially altering their function.
Biochemical Pathways
It’s known to be an intermediate in the synthesis of other compounds . This suggests that it may play a role in various biochemical reactions, potentially influencing multiple pathways.
Pharmacokinetics
It’s soluble in 1 m nh4oh, suggesting it may have good bioavailability .
Action Environment
The action of this compound may be influenced by various environmental factors. For instance, it’s known to be hygroscopic , suggesting that moisture in the environment could affect its stability and efficacy. Additionally, it’s recommended to store the compound under inert gas and in a cool, dark place , indicating that exposure to oxygen and light may impact its stability.
Biochemical Analysis
Biochemical Properties
4-Cyanopyridine N-oxide plays a significant role in biochemical reactions due to its ability to interact with various enzymes, proteins, and other biomolecules. It acts as a ligand for metals or other compounds, forming complexes that can influence biochemical pathways . For instance, it can coordinate with metal atoms, resulting in a linear bridge between two metal atoms . This interaction can affect the activity of enzymes and proteins involved in these pathways, thereby influencing the overall biochemical reactions.
Cellular Effects
The effects of this compound on various types of cells and cellular processes are profound. It influences cell function by affecting cell signaling pathways, gene expression, and cellular metabolism. The compound’s interaction with cellular components can lead to changes in the expression of specific genes, thereby altering cellular metabolism and function . Additionally, its role as a ligand can impact cell signaling pathways, leading to changes in cellular responses to external stimuli.
Molecular Mechanism
At the molecular level, this compound exerts its effects through binding interactions with biomolecules, enzyme inhibition or activation, and changes in gene expression. The compound can coordinate with metal atoms, forming complexes that can inhibit or activate specific enzymes . These interactions can lead to changes in the activity of these enzymes, thereby affecting the overall biochemical pathways. Additionally, the compound’s ability to influence gene expression can result in changes in cellular function and metabolism .
Temporal Effects in Laboratory Settings
In laboratory settings, the effects of this compound can change over time. The compound’s stability and degradation can influence its long-term effects on cellular function. Studies have shown that this compound is stable under certain conditions, but its degradation can lead to changes in its biochemical properties . Long-term exposure to the compound in in vitro or in vivo studies can result in alterations in cellular function, highlighting the importance of understanding its temporal effects.
Dosage Effects in Animal Models
The effects of this compound vary with different dosages in animal models. At lower doses, the compound may have minimal effects, while higher doses can lead to toxic or adverse effects . Studies have shown that there are threshold effects observed with different dosages, and it is crucial to determine the optimal dosage to minimize adverse effects while maximizing the compound’s beneficial properties.
Metabolic Pathways
This compound is involved in various metabolic pathways, interacting with enzymes and cofactors that influence metabolic flux and metabolite levels . The compound’s role as a ligand allows it to participate in biochemical reactions that can alter the levels of specific metabolites, thereby affecting overall metabolic pathways. Understanding these interactions is essential for elucidating the compound’s role in metabolism.
Transport and Distribution
Within cells and tissues, this compound is transported and distributed through interactions with transporters and binding proteins . These interactions can influence the compound’s localization and accumulation within specific cellular compartments. The compound’s ability to bind to specific proteins can affect its distribution and overall activity within the cell.
Subcellular Localization
The subcellular localization of this compound is crucial for its activity and function. The compound can be directed to specific compartments or organelles through targeting signals or post-translational modifications . These modifications can influence the compound’s activity and its interactions with other biomolecules, thereby affecting its overall function within the cell.
Preparation Methods
Synthetic Routes and Reaction Conditions: 4-Cyanopyridine N-oxide can be synthesized through the oxidation of 4-cyanopyridine using oxidizing agents such as hydrogen peroxide or peracids. One common method involves the use of titanium silicalite (TS-1) in a packed-bed microreactor with hydrogen peroxide in methanol as the solvent. This method is efficient and environmentally friendly, providing high yields of the desired product .
Industrial Production Methods: In industrial settings, the preparation of this compound often involves the use of sodium percarbonate as an oxygen source in the presence of rhenium-based catalysts. This method is preferred due to its mild reaction conditions and high efficiency .
Chemical Reactions Analysis
Types of Reactions: 4-Cyanopyridine N-oxide undergoes various types of chemical reactions, including:
Oxidation: It can be further oxidized to form other derivatives.
Reduction: The compound can be reduced to 4-cyanopyridine.
Substitution: It can participate in nucleophilic substitution reactions, where the cyano group or the N-oxide group can be replaced by other substituents.
Common Reagents and Conditions:
Oxidation: Hydrogen peroxide, peracids, and sodium percarbonate.
Reduction: Catalytic hydrogenation or the use of reducing agents like lithium aluminum hydride.
Substitution: Various nucleophiles under basic or acidic conditions.
Major Products Formed:
Oxidation: Further oxidized pyridine derivatives.
Reduction: 4-Cyanopyridine.
Substitution: Substituted pyridine derivatives depending on the nucleophile used.
Scientific Research Applications
4-Cyanopyridine N-oxide has a wide range of applications in scientific research:
Chemistry: It is used as a building block in the synthesis of more complex heterocyclic compounds.
Biology: The compound is studied for its potential biological activities and interactions with biomolecules.
Medicine: Research is ongoing to explore its potential as a pharmaceutical intermediate.
Industry: It is used in the production of various fine chemicals and as a ligand in coordination chemistry
Comparison with Similar Compounds
- 4-Methylpyridine N-oxide
- 4-Phenylpyridine N-oxide
- 4-Chloropyridine N-oxide
Comparison: 4-Cyanopyridine N-oxide is unique due to the presence of the cyano group, which imparts distinct electronic properties and reactivity compared to other pyridine N-oxides. The cyano group enhances the compound’s ability to participate in nucleophilic substitution reactions and influences its coordination behavior with metal ions .
Properties
IUPAC Name |
1-oxidopyridin-1-ium-4-carbonitrile | |
---|---|---|
Source | PubChem | |
URL | https://pubchem.ncbi.nlm.nih.gov | |
Description | Data deposited in or computed by PubChem | |
InChI |
InChI=1S/C6H4N2O/c7-5-6-1-3-8(9)4-2-6/h1-4H | |
Source | PubChem | |
URL | https://pubchem.ncbi.nlm.nih.gov | |
Description | Data deposited in or computed by PubChem | |
InChI Key |
QNCSFBSIWVBTHE-UHFFFAOYSA-N | |
Source | PubChem | |
URL | https://pubchem.ncbi.nlm.nih.gov | |
Description | Data deposited in or computed by PubChem | |
Canonical SMILES |
C1=C[N+](=CC=C1C#N)[O-] | |
Source | PubChem | |
URL | https://pubchem.ncbi.nlm.nih.gov | |
Description | Data deposited in or computed by PubChem | |
Molecular Formula |
C6H4N2O | |
Source | PubChem | |
URL | https://pubchem.ncbi.nlm.nih.gov | |
Description | Data deposited in or computed by PubChem | |
Molecular Weight |
120.11 g/mol | |
Source | PubChem | |
URL | https://pubchem.ncbi.nlm.nih.gov | |
Description | Data deposited in or computed by PubChem | |
CAS No. |
14906-59-3 | |
Record name | 4-Pyridinecarbonitrile, 1-oxide | |
Source | CAS Common Chemistry | |
URL | https://commonchemistry.cas.org/detail?cas_rn=14906-59-3 | |
Description | CAS Common Chemistry is an open community resource for accessing chemical information. Nearly 500,000 chemical substances from CAS REGISTRY cover areas of community interest, including common and frequently regulated chemicals, and those relevant to high school and undergraduate chemistry classes. This chemical information, curated by our expert scientists, is provided in alignment with our mission as a division of the American Chemical Society. | |
Explanation | The data from CAS Common Chemistry is provided under a CC-BY-NC 4.0 license, unless otherwise stated. | |
Record name | 4-Cyanopyridine N-oxide | |
Source | DTP/NCI | |
URL | https://dtp.cancer.gov/dtpstandard/servlet/dwindex?searchtype=NSC&outputformat=html&searchlist=132883 | |
Description | The NCI Development Therapeutics Program (DTP) provides services and resources to the academic and private-sector research communities worldwide to facilitate the discovery and development of new cancer therapeutic agents. | |
Explanation | Unless otherwise indicated, all text within NCI products is free of copyright and may be reused without our permission. Credit the National Cancer Institute as the source. | |
Record name | 4-Pyridinecarbonitrile, 1-oxide | |
Source | EPA Chemicals under the TSCA | |
URL | https://www.epa.gov/chemicals-under-tsca | |
Description | EPA Chemicals under the Toxic Substances Control Act (TSCA) collection contains information on chemicals and their regulations under TSCA, including non-confidential content from the TSCA Chemical Substance Inventory and Chemical Data Reporting. | |
Record name | Isonicotinonitrile 1-oxide | |
Source | European Chemicals Agency (ECHA) | |
URL | https://echa.europa.eu/substance-information/-/substanceinfo/100.035.416 | |
Description | The European Chemicals Agency (ECHA) is an agency of the European Union which is the driving force among regulatory authorities in implementing the EU's groundbreaking chemicals legislation for the benefit of human health and the environment as well as for innovation and competitiveness. | |
Explanation | Use of the information, documents and data from the ECHA website is subject to the terms and conditions of this Legal Notice, and subject to other binding limitations provided for under applicable law, the information, documents and data made available on the ECHA website may be reproduced, distributed and/or used, totally or in part, for non-commercial purposes provided that ECHA is acknowledged as the source: "Source: European Chemicals Agency, http://echa.europa.eu/". Such acknowledgement must be included in each copy of the material. ECHA permits and encourages organisations and individuals to create links to the ECHA website under the following cumulative conditions: Links can only be made to webpages that provide a link to the Legal Notice page. | |
Synthesis routes and methods I
Procedure details
Synthesis routes and methods II
Procedure details
Retrosynthesis Analysis
AI-Powered Synthesis Planning: Our tool employs the Template_relevance Pistachio, Template_relevance Bkms_metabolic, Template_relevance Pistachio_ringbreaker, Template_relevance Reaxys, Template_relevance Reaxys_biocatalysis model, leveraging a vast database of chemical reactions to predict feasible synthetic routes.
One-Step Synthesis Focus: Specifically designed for one-step synthesis, it provides concise and direct routes for your target compounds, streamlining the synthesis process.
Accurate Predictions: Utilizing the extensive PISTACHIO, BKMS_METABOLIC, PISTACHIO_RINGBREAKER, REAXYS, REAXYS_BIOCATALYSIS database, our tool offers high-accuracy predictions, reflecting the latest in chemical research and data.
Strategy Settings
Precursor scoring | Relevance Heuristic |
---|---|
Min. plausibility | 0.01 |
Model | Template_relevance |
Template Set | Pistachio/Bkms_metabolic/Pistachio_ringbreaker/Reaxys/Reaxys_biocatalysis |
Top-N result to add to graph | 6 |
Feasible Synthetic Routes
Q1: What is the molecular formula, weight, and spectroscopic data for 4-cyanopyridine N-oxide?
A1: this compound has the molecular formula C6H4N2O and a molecular weight of 120.11 g/mol []. The crystal structure of this compound was determined by X-ray diffraction, revealing that the 4-cyano substituent almost lies in the mean plane of the pyridine ring []. You can find more detailed spectroscopic data, including IR and NMR, in various research articles dedicated to this compound and its complexes.
Q2: How does this compound coordinate to metal ions?
A2: this compound can act as a ligand, coordinating to metal ions in different ways. It can coordinate through the oxygen atom of the N-oxide group, as seen in complexes with lanthanide trifluoroacetates [] and manganese(II) hexafluoroacetylacetonate []. Alternatively, it can coordinate through the nitrogen atom of the cyano group, as observed in complexes with cobalt(III) [, ].
Q3: Can you provide examples of metal complexes containing this compound and their potential applications?
A3: this compound forms complexes with various metals, including:
- Lanthanide Trifluoroacetates: These complexes exhibit lanthanide-centered luminescence upon UV excitation, with potential applications as solid-state light emitters [].
- Manganese(II) Complexes: One-dimensional polymeric structures have been synthesized, where manganese ions are bridged by dicyanamide ligands. These materials exhibit weak antiferromagnetic interactions [].
- Dimeric Lanthanide Hexafluoroacetylacetonate Adducts: These complexes exhibit metal-centered luminescence and have potential in fabricating emitting layers for light-emitting diodes [].
Q4: What is the role of this compound in the synthesis of tetrazoles?
A4: this compound serves as a precursor in the synthesis of 5-(4-pyridyl N-oxide)tetrazole (4-HPTZ) through a [2+3] cycloaddition reaction with sodium azide (NaN3) []. Cadmium chloride (CdCl2) can be used as a catalyst in this reaction, forming an intermediate complex, [Cd(4-PTZ)2(H2O)4], which further dissociates to release the desired tetrazole product [].
Q5: Has this compound been investigated for catalytic activity?
A5: While not a catalyst itself, this compound plays a crucial role in understanding catalytic processes. It acts as a hole scavenger in photoelectrochemical water oxidation studies using WO3 photoanodes []. By suppressing oxygen formation, it helps researchers understand the competition between water and anion oxidation at the electrode surface.
Disclaimer and Information on In-Vitro Research Products
Please be aware that all articles and product information presented on BenchChem are intended solely for informational purposes. The products available for purchase on BenchChem are specifically designed for in-vitro studies, which are conducted outside of living organisms. In-vitro studies, derived from the Latin term "in glass," involve experiments performed in controlled laboratory settings using cells or tissues. It is important to note that these products are not categorized as medicines or drugs, and they have not received approval from the FDA for the prevention, treatment, or cure of any medical condition, ailment, or disease. We must emphasize that any form of bodily introduction of these products into humans or animals is strictly prohibited by law. It is essential to adhere to these guidelines to ensure compliance with legal and ethical standards in research and experimentation.