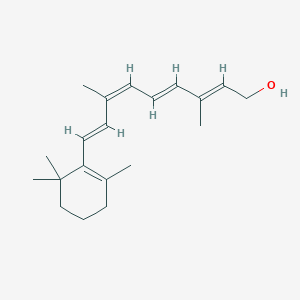
9-cis-Retinol
Overview
Description
9-cis-Retinol is a bioactive isomer of retinol (vitamin A alcohol) that serves as a critical precursor in the biosynthesis of 9-cis-retinoic acid (9cRA), a ligand for retinoid X receptors (RXRs) . Unlike all-trans-retinoic acid (ATRA), which primarily activates retinoic acid receptors (RARs), 9cRA regulates gene expression through RXR heterodimers, influencing processes such as embryonic development, cell differentiation, and lipid metabolism . The conversion of this compound to 9cRA involves two enzymatic steps: oxidation to 9-cis-retinaldehyde by this compound dehydrogenase (9cRDH) and subsequent oxidation to 9cRA by retinal dehydrogenases (RALDHs) .
Key enzymatic pathways for this compound metabolism are tissue-specific. Additionally, this compound can be esterified by acyltransferases or stored as retinyl esters, which are hydrolyzed during metabolic demand .
Preparation Methods
Biosynthetic Pathways of 9-cis-Retinol
Enzymatic Conversion from 9-cis-β-Carotene
The intestinal mucosa facilitates the cleavage of 9-cis-β-carotene into 9-cis-retinal via β-carotene oxygenase 1 (BCO1), followed by reduction to 9-cis-retinol through retinol dehydrogenases . In vitro studies using human intestinal homogenates demonstrated a conversion rate of 16 ± 1 pmol/hr/mg protein for 9-cis-retinoic acid when incubated with 4 µM 9-cis-β-carotene . Notably, co-incubation with all-trans-β-carotene doubled the yield of all-trans-retinoic acid (38 ± 6 pmol/hr/mg protein) without affecting 9-cis-retinol synthesis, suggesting compartmentalized enzymatic activity .
Retinal Isomerization Pathways
Alternative pathways involve the isomerization of all-trans-retinal to 9-cis-retinal via retinal isomerases, though this route is less efficient (<5% yield) . Oxidative conversion of 9-cis-retinal to 9-cis-retinoic acid further complicates isolation, necessitating precise redox control during biosynthesis .
Synthetic Production from Algal Sources
Extraction from Dunaliella bardawil
Dunaliella bardawil, a halophilic microalga, accumulates 9-cis-β-carotene as a stress response to high salinity and light . Ethanol-based extraction yields 9-cis-β-carotene at 30–40 mg/g dry biomass, which is subsequently cleaved using recombinant BCO1 to produce 9-cis-retinal . Catalytic hydrogenation with palladium on carbon (Pd/C) under mild conditions (25°C, 1 atm H₂) achieves >90% reduction to 9-cis-retinol .
Table 1: Algal-Derived this compound Production Metrics
Parameter | Value |
---|---|
9-cis-β-carotene yield | 30–40 mg/g dry biomass |
BCO1 cleavage efficiency | 85–92% |
Hydrogenation yield | 90–95% |
Purity (HPLC) | ≥98% |
Chemical Synthesis from β-Ionone Precursors
Multi-Step Synthesis via Conjugate Addition
A patented six-step route synthesizes 9-cis-retinol from β-ionone with 22% overall yield :
-
Alkyne Formation : β-Ionone undergoes alkyne coupling with propargyl bromide to form 4-(2,6,6-trimethylcyclohexenyl)-but-3-ene-1-yne .
-
Cyanoenolate Addition : Treatment with lithium diisopropylamide (LDA) and acrylonitrile generates a cyanoenolate intermediate .
-
Conjugate Alkylation : Methyl cuprate addition ensures cis-configuration at C9–C10 .
-
Reduction : Diisobutylaluminum hydride (DIBAL-H) reduces nitriles to aldehydes .
-
Wittig Olefination : Reaction with ethyl (triphenylphosphoranylidene)acetate extends the polyene chain .
-
Saponification : Hydrolysis with KOH/MeOH yields 9-cis-retinoic acid, followed by borohydride reduction to retinol .
Stereochemical Control
The cis-selectivity at C9–C10 is achieved through steric hindrance during the conjugate alkylation step, where methyl cuprate adds to the less hindered face of the cyanoenolate intermediate . Polar solvents like tetrahydrofuran (THF) enhance regioselectivity (>95% cis) .
Table 2: Key Reaction Parameters in Chemical Synthesis
Step | Reagents/Conditions | Yield |
---|---|---|
Alkyne formation | Propargyl bromide, KOtBu | 78% |
Cyanoenolate addition | LDA, acrylonitrile, −78°C | 85% |
Conjugate alkylation | Methyl cuprate, −30°C | 90% |
Wittig olefination | Ph₃P=CHCO₂Et, DMF, 25°C | 75% |
Purification and Characterization
Chromatographic Separation
Reverse-phase HPLC (C18 column, 70% acetonitrile/water) resolves 9-cis-retinol from all-trans contaminants with a retention time of 12.3 min . Preparative TLC (silica gel, hexane:acetone 8:2) achieves >99% purity for small-scale batches .
Spectroscopic Confirmation
Comparative Analysis of Preparation Methods
Table 3: Method Comparison
Method | Yield | Cost | Scalability | Cis-Purity |
---|---|---|---|---|
Biosynthesis | 15–18% | High | Low | 85–90% |
Algal extraction | 25–30% | Medium | Moderate | 92–95% |
Chemical synthesis | 20–22% | Low | High | 98–99% |
Chemical Reactions Analysis
- Zuretinol acetate likely undergoes various chemical reactions, including oxidation, reduction, and substitution.
- Common reagents and conditions used in these reactions remain undisclosed.
- The major products formed from these reactions are not explicitly documented.
Scientific Research Applications
Vision Science
1.1 Retinal Health and Function
Research indicates that 9-cis-Retinol plays a crucial role in the regeneration of photoreceptors and the maintenance of visual function. A significant study demonstrated that long-term administration of 9-cis-retinyl acetate (a prodrug of this compound) improved rod and cone visual functions in mice. The study found that this treatment enhanced the regeneration ratio of rhodopsin and improved electroretinographic (ERG) responses, suggesting potential therapeutic benefits for age-related retinal degeneration .
Table 1: Effects of 9-cis-Retinyl Acetate on Retinal Function
Parameter | Control Group | 9-cis-Retinyl Acetate Group |
---|---|---|
Rhodopsin Regeneration Ratio | Decreased | Increased |
ERG Responses | Decreased | Improved |
Dark Adaptation | Impaired | Enhanced |
1.2 Treatment for Retinal Dystrophies
Another application involves the use of synthetic 9-cis-beta-carotene as a treatment for retinal dystrophies. This compound can replace the missing native chromophore in rhodopsin, forming light-sensitive isorhodopsin in vivo. Clinical studies have shown that oral treatment with 9-cis-retinyl acetate significantly improved retinal function in patients with Leber Congenital Amaurosis (LCA) and other genetic retinal disorders .
Dermatological Applications
2.1 Skin Differentiation and Proliferation
In dermatological research, this compound has been shown to modulate skin cell differentiation and proliferation. Studies indicate that treatment with 9-cis-retinoic acid can induce a more proliferative phenotype in human keratinocytes, affecting the expression of various proteins involved in skin health . This suggests potential applications in treating skin disorders or enhancing skin repair mechanisms.
Table 2: Effects on Keratinocyte Behavior
Treatment | Proliferation Rate | Involucrin Expression |
---|---|---|
Control | Baseline | Normal |
9-cis-Retinoic Acid | Increased | Delayed |
Cancer Research
3.1 Modulation of Cell Differentiation
The application of this compound in cancer research is notable for its ability to influence cell differentiation. It has been observed that this compound can induce differentiation in acute promyelocytic leukemia cells, presenting a potential therapeutic avenue for hematological malignancies .
Case Study: Acute Myeloid Leukemia
A study focused on the effects of 9-cis-retinoic acid on acute myeloid leukemia cells showed significant modulation of differentiation markers, suggesting its role as a differentiating agent in cancer therapy .
Mechanistic Insights
The mechanisms underlying the effects of this compound involve its interaction with retinoid receptors, particularly retinoid X receptors (RXRs). These receptors are critical for mediating the biological effects of retinoids, influencing gene expression related to vision, skin health, and cellular differentiation.
Mechanism of Action
- The precise mechanism by which Zuretinol acetate exerts its effects is not fully elucidated.
- It likely targets retinal pathways involved in visual function and photoreceptor health.
Comparison with Similar Compounds
Enzymatic Specificity and Catalytic Efficiency
Table 1: Substrate Specificity and Catalytic Activity of Retinol Dehydrogenases
- CRAD3 discriminates against all-trans-retinol but efficiently oxidizes this compound, with catalytic efficiency 3 orders of magnitude higher than RDH4 .
Binding Protein Affinities
Table 2: Binding Affinities of Retinol-Binding Proteins (KD Values)
- Key Findings: CRBPI binds this compound with ~3x lower affinity than all-trans-retinol but still facilitates its metabolism in tissues like liver and kidney . CRBPII shows minimal affinity for this compound, aligning with its role in intestinal uptake, where all-trans-retinol is the primary transported form .
Metabolic Pathways and Physiological Roles
Tissue-Specific Metabolism
- Intestine: All-trans-retinol absorption is facilitated by a specific transporter, while this compound uptake is passive and inefficient .
Pharmacological and Clinical Implications
- Inhibition: 13-cis-retinoic acid (isotretinoin) inhibits 9cRDH and CRAD3 by ~50% at 0.15 µM, suggesting therapeutic cross-reactivity .
- Disease Links: Elevated this compound levels in prostate tissue correlate with lycopene isomers, hinting at antioxidant roles in cancer prevention .
Biological Activity
9-cis-Retinol, a derivative of vitamin A, plays a significant role in various biological processes, particularly in vision, cellular differentiation, and growth regulation. This article explores the biological activity of this compound, focusing on its mechanisms of action, effects on cellular processes, and implications for health and disease.
Chemical Structure and Metabolism
This compound is an isomer of all-trans-retinol and serves as a precursor to 9-cis-retinoic acid (9-cis-RA), which activates retinoid receptors in the body. The conversion of this compound to 9-cis-RA is facilitated by retinol dehydrogenases, enzymes that play a critical role in retinoid metabolism. Research indicates that the efficient formation of 9-cis-RA requires the co-expression of specific dehydrogenases, highlighting the complexity of retinoid biosynthesis .
Table 1: Comparison of Retinoid Isomers
Compound | Structure | Biological Activity |
---|---|---|
All-trans-Retinol | Straight chain | Vision, cell differentiation |
This compound | Cis configuration | Modulates gene expression, affects insulin secretion |
Cellular Differentiation and Proliferation
Studies have shown that 9-cis-RA influences spermatogenesis in vitamin A-deficient mice. Administration of 9-cis-RA stimulated the differentiation and proliferation of growth-arrested A spermatogonia, although higher doses were required compared to all-trans-retinoic acid (ATRA). Interestingly, the presence of 9-cis-RA reduced the effectiveness of ATRA when both were administered simultaneously . This suggests that while both compounds share similar pathways, they may exert distinct effects on cellular processes.
Insulin Secretion Regulation
Recent research has identified 9-cis-RA as a pancreas-specific autacoid that modulates insulin secretion. In mouse islets and rat β-cell lines, 9-cis-RA inhibited glucose-stimulated insulin secretion (GSIS) by affecting glucose transporter type 2 levels. This action indicates a potential role for 9-cis-RA in glucose metabolism and diabetes management .
Gene Expression Modulation
The impact of this compound on gene expression has been noted in various studies. For instance, it transiently induces the expression of nuclear retinoic acid receptor RARβ, which is crucial for mediating retinoid effects on cellular differentiation . However, it does not significantly alter the expression levels of retinoid X receptors (RXRs), suggesting that its mechanisms may differ from those mediated by other retinoids .
Case Study: Spermatogenesis in Vitamin A Deficiency
A study involving vitamin A-deficient mice demonstrated that treatment with 9-cis-RA led to significant improvements in spermatogenesis. The results indicated that while both 9-cis-RA and ATRA promote spermatogonial proliferation, their efficacy varies based on dosage and timing. This highlights the potential therapeutic applications of 9-cis-RA in reproductive health .
Case Study: Insulin Secretion Dynamics
Another investigation into the role of 9-cis-RA in pancreatic function revealed its inhibitory effect on insulin secretion under high glucose conditions. This finding suggests a nuanced role for retinoids in metabolic regulation, particularly concerning energy homeostasis and diabetes management .
Q & A
Basic Research Questions
Q. How can researchers determine the binding affinities of 9-cis-retinol to cellular retinol-binding proteins (CRBPI and CRBPII)?
Q. What enzymatic pathways are involved in the oxidation of this compound to 9-cis-retinoic acid?
The conversion requires two steps: (1) oxidation of 9-cis-retinol to 9-cis-retinal by a stereospecific dehydrogenase (e.g., cis-retinol dehydrogenase, cRDH), and (2) further oxidation to 9-cis-retinoic acid via retinaldehyde dehydrogenases. Notably, RDH10, a key enzyme in all-trans-retinol metabolism, shows no activity toward 9-cis-retinol, indicating distinct pathways for isomer-specific retinoid processing .
Q. What experimental strategies are recommended for handling and solubilizing this compound in vitro?
9-cis-Retinol is light- and oxygen-sensitive. Use amber vials and inert gas purging during storage (-80°C). For solubility, dissolve in organic solvents (e.g., ethanol, DMSO) at concentrations ≤25 mg/mL, then dilute in aqueous buffers. Avoid prolonged storage of aqueous solutions (>24 hours) due to isomerization risks .
Advanced Research Questions
Q. How do conflicting data on CRBPI/II binding affinities for this compound versus other isomers inform retinoid transport mechanisms?
CRBPI binds 9-cis-retinol with ~3-fold lower affinity compared to all-trans- and 13-cis-retinol, while CRBPII exhibits a hierarchy: all-trans >13-cis >9-cis. These differences suggest compartmentalized roles: CRBPI may prioritize all-trans-retinoids for systemic storage, whereas CRBPII could fine-tune intestinal absorption of specific isomers . Structural studies (e.g., X-ray crystallography) are needed to resolve steric factors influencing isomer selectivity.
Q. Why does this compound fail to act as a substrate for RDH10, and what alternative enzymes mediate its oxidation?
RDH10’s catalytic pocket is sterically restricted to all-trans-retinol, as shown by mutagenesis and activity assays. Instead, membrane-bound cis-retinol dehydrogenases (e.g., cRDH) selectively oxidize 9-cis-retinol to 9-cis-retinal, a precursor for 9-cis-retinoic acid biosynthesis. This enzyme is highly expressed in embryonic tissues, aligning with 9-cis-retinoic acid’s role in developmental signaling .
Q. How can researchers design experiments to track this compound metabolic flux in vivo?
Isotopic labeling (e.g., 9-cis-retinol-) combined with LC-MS/MS allows quantification of metabolites in tissue extracts. For spatial resolution, use transgenic models (e.g., Rdh10 knockouts) or inhibitors to block competing pathways. In zebrafish, rdh10a mutants show disrupted retinoic acid signaling, providing a model to study 9-cis-retinol’s compensatory roles .
Q. What explains the isomer-specific modulation of STRA6-mediated retinol transport by this compound?
STRA6, a membrane receptor for retinol-binding protein (RBP), exhibits reduced sensitivity to 9-cis-retinol compared to all-trans-retinol. Competitive assays show 9-cis-retinol weakly inhibits STRA6-dependent retinol release from holo-RBP, suggesting stereochemical constraints in ligand-receptor interactions. This has implications for tissue-specific retinoid uptake and signaling .
Q. Methodological Considerations
Q. How should researchers validate the specificity of antibodies targeting retinoid-metabolizing enzymes like RDH10?
Use knockout cell lines or tissues (e.g., Rdh10 mice) in Western blotting to confirm antibody specificity. For functional validation, pair immunodetection with enzymatic assays (e.g., NADP-dependent retinol oxidation) and negative controls (e.g., 9-cis-retinol as a non-substrate) .
Q. What analytical techniques are optimal for quantifying this compound in complex biological matrices?
Reverse-phase HPLC with UV/Vis detection (λ = 325–373 nm) provides baseline separation from all-trans-retinol. For enhanced sensitivity, employ mass spectrometry (e.g., ESI-MS/MS) with deuterated internal standards (e.g., 9-cis-retinol-) to correct for isomerization artifacts during extraction .
Q. Contradiction Analysis
Q. How can conflicting reports on this compound’s role in gene transcription be reconciled?
While 9-cis-retinoic acid is a known RXR agonist, 9-cis-retinol itself may indirectly modulate transcription via metabolic conversion. Discrepancies arise from cell type-specific enzyme expression (e.g., cRDH vs. RDH10) and assay conditions (e.g., serum-free media reduces retinoid-binding protein interference). Use CRISPR-edited cell lines to isolate 9-cis-retinol’s direct effects .
Properties
IUPAC Name |
(2E,4E,6Z,8E)-3,7-dimethyl-9-(2,6,6-trimethylcyclohexen-1-yl)nona-2,4,6,8-tetraen-1-ol | |
---|---|---|
Source | PubChem | |
URL | https://pubchem.ncbi.nlm.nih.gov | |
Description | Data deposited in or computed by PubChem | |
InChI |
InChI=1S/C20H30O/c1-16(8-6-9-17(2)13-15-21)11-12-19-18(3)10-7-14-20(19,4)5/h6,8-9,11-13,21H,7,10,14-15H2,1-5H3/b9-6+,12-11+,16-8-,17-13+ | |
Source | PubChem | |
URL | https://pubchem.ncbi.nlm.nih.gov | |
Description | Data deposited in or computed by PubChem | |
InChI Key |
FPIPGXGPPPQFEQ-MKOSUFFBSA-N | |
Source | PubChem | |
URL | https://pubchem.ncbi.nlm.nih.gov | |
Description | Data deposited in or computed by PubChem | |
Canonical SMILES |
CC1=C(C(CCC1)(C)C)C=CC(=CC=CC(=CCO)C)C | |
Source | PubChem | |
URL | https://pubchem.ncbi.nlm.nih.gov | |
Description | Data deposited in or computed by PubChem | |
Isomeric SMILES |
CC1=C(C(CCC1)(C)C)/C=C/C(=C\C=C\C(=C\CO)\C)/C | |
Source | PubChem | |
URL | https://pubchem.ncbi.nlm.nih.gov | |
Description | Data deposited in or computed by PubChem | |
Molecular Formula |
C20H30O | |
Source | PubChem | |
URL | https://pubchem.ncbi.nlm.nih.gov | |
Description | Data deposited in or computed by PubChem | |
DSSTOX Substance ID |
DTXSID201317690 | |
Record name | 9-cis-Retinol | |
Source | EPA DSSTox | |
URL | https://comptox.epa.gov/dashboard/DTXSID201317690 | |
Description | DSSTox provides a high quality public chemistry resource for supporting improved predictive toxicology. | |
Molecular Weight |
286.5 g/mol | |
Source | PubChem | |
URL | https://pubchem.ncbi.nlm.nih.gov | |
Description | Data deposited in or computed by PubChem | |
Physical Description |
Solid | |
Record name | 9-cis-Retinol | |
Source | Human Metabolome Database (HMDB) | |
URL | http://www.hmdb.ca/metabolites/HMDB0006217 | |
Description | The Human Metabolome Database (HMDB) is a freely available electronic database containing detailed information about small molecule metabolites found in the human body. | |
Explanation | HMDB is offered to the public as a freely available resource. Use and re-distribution of the data, in whole or in part, for commercial purposes requires explicit permission of the authors and explicit acknowledgment of the source material (HMDB) and the original publication (see the HMDB citing page). We ask that users who download significant portions of the database cite the HMDB paper in any resulting publications. | |
CAS No. |
22737-97-9 | |
Record name | 9-cis-Retinol | |
Source | CAS Common Chemistry | |
URL | https://commonchemistry.cas.org/detail?cas_rn=22737-97-9 | |
Description | CAS Common Chemistry is an open community resource for accessing chemical information. Nearly 500,000 chemical substances from CAS REGISTRY cover areas of community interest, including common and frequently regulated chemicals, and those relevant to high school and undergraduate chemistry classes. This chemical information, curated by our expert scientists, is provided in alignment with our mission as a division of the American Chemical Society. | |
Explanation | The data from CAS Common Chemistry is provided under a CC-BY-NC 4.0 license, unless otherwise stated. | |
Record name | Zuretinol | |
Source | ChemIDplus | |
URL | https://pubchem.ncbi.nlm.nih.gov/substance/?source=chemidplus&sourceid=0022737979 | |
Description | ChemIDplus is a free, web search system that provides access to the structure and nomenclature authority files used for the identification of chemical substances cited in National Library of Medicine (NLM) databases, including the TOXNET system. | |
Record name | 9-cis-Retinol | |
Source | EPA DSSTox | |
URL | https://comptox.epa.gov/dashboard/DTXSID201317690 | |
Description | DSSTox provides a high quality public chemistry resource for supporting improved predictive toxicology. | |
Record name | ZURETINOL | |
Source | FDA Global Substance Registration System (GSRS) | |
URL | https://gsrs.ncats.nih.gov/ginas/app/beta/substances/035JKP3HXH | |
Description | The FDA Global Substance Registration System (GSRS) enables the efficient and accurate exchange of information on what substances are in regulated products. Instead of relying on names, which vary across regulatory domains, countries, and regions, the GSRS knowledge base makes it possible for substances to be defined by standardized, scientific descriptions. | |
Explanation | Unless otherwise noted, the contents of the FDA website (www.fda.gov), both text and graphics, are not copyrighted. They are in the public domain and may be republished, reprinted and otherwise used freely by anyone without the need to obtain permission from FDA. Credit to the U.S. Food and Drug Administration as the source is appreciated but not required. | |
Record name | 9-cis-Retinol | |
Source | Human Metabolome Database (HMDB) | |
URL | http://www.hmdb.ca/metabolites/HMDB0006217 | |
Description | The Human Metabolome Database (HMDB) is a freely available electronic database containing detailed information about small molecule metabolites found in the human body. | |
Explanation | HMDB is offered to the public as a freely available resource. Use and re-distribution of the data, in whole or in part, for commercial purposes requires explicit permission of the authors and explicit acknowledgment of the source material (HMDB) and the original publication (see the HMDB citing page). We ask that users who download significant portions of the database cite the HMDB paper in any resulting publications. | |
Retrosynthesis Analysis
AI-Powered Synthesis Planning: Our tool employs the Template_relevance Pistachio, Template_relevance Bkms_metabolic, Template_relevance Pistachio_ringbreaker, Template_relevance Reaxys, Template_relevance Reaxys_biocatalysis model, leveraging a vast database of chemical reactions to predict feasible synthetic routes.
One-Step Synthesis Focus: Specifically designed for one-step synthesis, it provides concise and direct routes for your target compounds, streamlining the synthesis process.
Accurate Predictions: Utilizing the extensive PISTACHIO, BKMS_METABOLIC, PISTACHIO_RINGBREAKER, REAXYS, REAXYS_BIOCATALYSIS database, our tool offers high-accuracy predictions, reflecting the latest in chemical research and data.
Strategy Settings
Precursor scoring | Relevance Heuristic |
---|---|
Min. plausibility | 0.01 |
Model | Template_relevance |
Template Set | Pistachio/Bkms_metabolic/Pistachio_ringbreaker/Reaxys/Reaxys_biocatalysis |
Top-N result to add to graph | 6 |
Feasible Synthetic Routes
Disclaimer and Information on In-Vitro Research Products
Please be aware that all articles and product information presented on BenchChem are intended solely for informational purposes. The products available for purchase on BenchChem are specifically designed for in-vitro studies, which are conducted outside of living organisms. In-vitro studies, derived from the Latin term "in glass," involve experiments performed in controlled laboratory settings using cells or tissues. It is important to note that these products are not categorized as medicines or drugs, and they have not received approval from the FDA for the prevention, treatment, or cure of any medical condition, ailment, or disease. We must emphasize that any form of bodily introduction of these products into humans or animals is strictly prohibited by law. It is essential to adhere to these guidelines to ensure compliance with legal and ethical standards in research and experimentation.