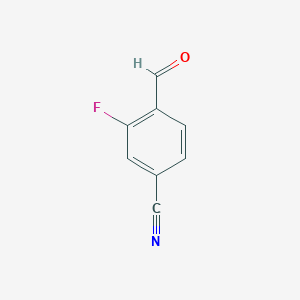
4-Cyano-2-fluorobenzaldehyde
Overview
Description
4-Cyano-2-fluorobenzaldehyde is an organic compound characterized by the presence of cyano and fluorine functional groups. It has the chemical formula C8H4FNO and a molecular weight of 149.12 g/mol . This compound is a pale yellow solid and is commonly used as a building block in the synthesis of pharmaceuticals and agrochemicals .
Mechanism of Action
Target of Action
It’s known that similar compounds, such as fluorobenzaldehydes, are often used as synthetic intermediates . They can be used to make a variety of Schiff base compounds through a condensation reaction , some of which have antimicrobial properties .
Mode of Action
It’s known that fluorobenzaldehydes can undergo a variety of chemical reactions due to the presence of the aldehyde group . For instance, they can participate in condensation reactions to form Schiff base compounds . These reactions involve the interaction of the aldehyde group with a primary amine to form a Schiff base, a compound with a carbon-nitrogen double bond .
Biochemical Pathways
Schiff base compounds, which can be synthesized from fluorobenzaldehydes, have been found to exhibit antimicrobial properties . This suggests that they may interact with biochemical pathways in microorganisms, potentially inhibiting essential enzymatic reactions or disrupting cell wall synthesis.
Pharmacokinetics
For instance, fluorobenzaldehydes have relatively low molecular weights and appropriate lipophilicity, which are favorable properties for absorption and distribution .
Result of Action
The antimicrobial properties of schiff base compounds synthesized from similar compounds suggest that they may disrupt essential cellular processes in microorganisms .
Biochemical Analysis
Biochemical Properties
4-Cyano-2-fluorobenzaldehyde plays a significant role in biochemical reactions, particularly in the synthesis of Schiff base compounds through condensation reactions. These Schiff base compounds have been found to exhibit antimicrobial properties . The aldehyde group in this compound allows it to interact with various enzymes and proteins, facilitating the formation of these bioactive compounds. Additionally, the fluorine atom in the compound can be replaced via oxidation reactions, further expanding its utility in biochemical synthesis .
Cellular Effects
This compound has been shown to influence various cellular processes. It can affect cell signaling pathways, gene expression, and cellular metabolism. The compound’s interaction with cellular proteins and enzymes can lead to changes in cell function, potentially impacting cell growth and proliferation. Studies have indicated that this compound can induce oxidative stress in cells, leading to alterations in cellular homeostasis .
Molecular Mechanism
The molecular mechanism of this compound involves its interaction with biomolecules at the molecular level. The compound can bind to specific enzymes and proteins, leading to enzyme inhibition or activation. This binding interaction can result in changes in gene expression and subsequent cellular responses. For example, the compound’s aldehyde group can form covalent bonds with amino acid residues in proteins, altering their structure and function .
Temporal Effects in Laboratory Settings
In laboratory settings, the effects of this compound can change over time. The compound’s stability and degradation are important factors to consider. Studies have shown that this compound is relatively stable under standard laboratory conditions, but it can degrade over time, leading to changes in its biochemical activity. Long-term exposure to the compound can result in cumulative effects on cellular function, including potential cytotoxicity .
Dosage Effects in Animal Models
The effects of this compound vary with different dosages in animal models. At low doses, the compound may exhibit minimal toxicity and can be used to study its biochemical properties. At higher doses, this compound can induce toxic effects, including oxidative stress and cellular damage. Threshold effects have been observed, where the compound’s impact on cellular function becomes more pronounced at specific dosage levels .
Metabolic Pathways
This compound is involved in various metabolic pathways. The compound can undergo oxidation reactions, leading to the formation of different metabolites. Enzymes such as cytochrome P450 oxidases play a crucial role in the metabolism of this compound. These metabolic pathways can influence the compound’s bioavailability and overall biochemical activity .
Transport and Distribution
Within cells and tissues, this compound is transported and distributed through specific transporters and binding proteins. The compound’s interaction with these transporters can affect its localization and accumulation within different cellular compartments. Understanding the transport and distribution of this compound is essential for elucidating its biochemical effects and potential therapeutic applications .
Subcellular Localization
The subcellular localization of this compound can influence its activity and function. The compound may be directed to specific cellular compartments or organelles through targeting signals or post-translational modifications. This localization can impact the compound’s interaction with biomolecules and its overall biochemical activity. Studies have shown that this compound can accumulate in the cytoplasm and nucleus, affecting various cellular processes .
Preparation Methods
4-Cyano-2-fluorobenzaldehyde can be synthesized through various methods. One common synthetic route involves the halogen-exchange reaction with 4-chlorobenzaldehyde . Another method includes the use of 3,4-difluorobenzonitrile as a starting material . Industrial production methods often involve the use of specific catalysts and conditions to introduce the fluorine atom into the benzaldehyde structure.
Chemical Reactions Analysis
4-Cyano-2-fluorobenzaldehyde undergoes various chemical reactions, including:
Oxidation: The compound can be oxidized to form different products depending on the reagents and conditions used.
Reduction: Reduction reactions can convert the aldehyde group to an alcohol.
Substitution: The fluorine atom can be substituted with different groups under various conditions, including nucleophilic substitution reactions.
Condensation: The compound can form Schiff bases through reactions with amino acids in refluxing ethanol.
Common reagents used in these reactions include potassium fluoride, specific catalysts, and ethanol. Major products formed from these reactions include Schiff bases and other substituted benzaldehyde derivatives.
Scientific Research Applications
4-Cyano-2-fluorobenzaldehyde has a wide range of scientific research applications:
Chemistry: It is used as a synthetic intermediate in the preparation of various organic molecules.
Industry: The compound is used in the production of agrochemicals and other industrial chemicals.
Comparison with Similar Compounds
4-Cyano-2-fluorobenzaldehyde can be compared with other fluorinated benzaldehyde derivatives, such as:
- 2-Fluorobenzaldehyde
- 3-Fluorobenzaldehyde
- 4-Fluorobenzaldehyde
These compounds differ in the location of the fluorine atom on the benzaldehyde ring but share similar chemical properties .
Properties
IUPAC Name |
3-fluoro-4-formylbenzonitrile | |
---|---|---|
Source | PubChem | |
URL | https://pubchem.ncbi.nlm.nih.gov | |
Description | Data deposited in or computed by PubChem | |
InChI |
InChI=1S/C8H4FNO/c9-8-3-6(4-10)1-2-7(8)5-11/h1-3,5H | |
Source | PubChem | |
URL | https://pubchem.ncbi.nlm.nih.gov | |
Description | Data deposited in or computed by PubChem | |
InChI Key |
QXHUSGWCFSXQMF-UHFFFAOYSA-N | |
Source | PubChem | |
URL | https://pubchem.ncbi.nlm.nih.gov | |
Description | Data deposited in or computed by PubChem | |
Canonical SMILES |
C1=CC(=C(C=C1C#N)F)C=O | |
Source | PubChem | |
URL | https://pubchem.ncbi.nlm.nih.gov | |
Description | Data deposited in or computed by PubChem | |
Molecular Formula |
C8H4FNO | |
Source | PubChem | |
URL | https://pubchem.ncbi.nlm.nih.gov | |
Description | Data deposited in or computed by PubChem | |
DSSTOX Substance ID |
DTXSID90382463 | |
Record name | 4-Cyano-2-fluorobenzaldehyde | |
Source | EPA DSSTox | |
URL | https://comptox.epa.gov/dashboard/DTXSID90382463 | |
Description | DSSTox provides a high quality public chemistry resource for supporting improved predictive toxicology. | |
Molecular Weight |
149.12 g/mol | |
Source | PubChem | |
URL | https://pubchem.ncbi.nlm.nih.gov | |
Description | Data deposited in or computed by PubChem | |
CAS No. |
105942-10-7 | |
Record name | 3-Fluoro-4-formylbenzonitrile | |
Source | CAS Common Chemistry | |
URL | https://commonchemistry.cas.org/detail?cas_rn=105942-10-7 | |
Description | CAS Common Chemistry is an open community resource for accessing chemical information. Nearly 500,000 chemical substances from CAS REGISTRY cover areas of community interest, including common and frequently regulated chemicals, and those relevant to high school and undergraduate chemistry classes. This chemical information, curated by our expert scientists, is provided in alignment with our mission as a division of the American Chemical Society. | |
Explanation | The data from CAS Common Chemistry is provided under a CC-BY-NC 4.0 license, unless otherwise stated. | |
Record name | 4-Cyano-2-fluorobenzaldehyde | |
Source | EPA DSSTox | |
URL | https://comptox.epa.gov/dashboard/DTXSID90382463 | |
Description | DSSTox provides a high quality public chemistry resource for supporting improved predictive toxicology. | |
Record name | 3-fluoro-4-formylbenzonitrile | |
Source | European Chemicals Agency (ECHA) | |
URL | https://echa.europa.eu/information-on-chemicals | |
Description | The European Chemicals Agency (ECHA) is an agency of the European Union which is the driving force among regulatory authorities in implementing the EU's groundbreaking chemicals legislation for the benefit of human health and the environment as well as for innovation and competitiveness. | |
Explanation | Use of the information, documents and data from the ECHA website is subject to the terms and conditions of this Legal Notice, and subject to other binding limitations provided for under applicable law, the information, documents and data made available on the ECHA website may be reproduced, distributed and/or used, totally or in part, for non-commercial purposes provided that ECHA is acknowledged as the source: "Source: European Chemicals Agency, http://echa.europa.eu/". Such acknowledgement must be included in each copy of the material. ECHA permits and encourages organisations and individuals to create links to the ECHA website under the following cumulative conditions: Links can only be made to webpages that provide a link to the Legal Notice page. | |
Synthesis routes and methods I
Procedure details
Synthesis routes and methods II
Procedure details
Synthesis routes and methods III
Procedure details
Synthesis routes and methods IV
Procedure details
Retrosynthesis Analysis
AI-Powered Synthesis Planning: Our tool employs the Template_relevance Pistachio, Template_relevance Bkms_metabolic, Template_relevance Pistachio_ringbreaker, Template_relevance Reaxys, Template_relevance Reaxys_biocatalysis model, leveraging a vast database of chemical reactions to predict feasible synthetic routes.
One-Step Synthesis Focus: Specifically designed for one-step synthesis, it provides concise and direct routes for your target compounds, streamlining the synthesis process.
Accurate Predictions: Utilizing the extensive PISTACHIO, BKMS_METABOLIC, PISTACHIO_RINGBREAKER, REAXYS, REAXYS_BIOCATALYSIS database, our tool offers high-accuracy predictions, reflecting the latest in chemical research and data.
Strategy Settings
Precursor scoring | Relevance Heuristic |
---|---|
Min. plausibility | 0.01 |
Model | Template_relevance |
Template Set | Pistachio/Bkms_metabolic/Pistachio_ringbreaker/Reaxys/Reaxys_biocatalysis |
Top-N result to add to graph | 6 |
Feasible Synthetic Routes
Disclaimer and Information on In-Vitro Research Products
Please be aware that all articles and product information presented on BenchChem are intended solely for informational purposes. The products available for purchase on BenchChem are specifically designed for in-vitro studies, which are conducted outside of living organisms. In-vitro studies, derived from the Latin term "in glass," involve experiments performed in controlled laboratory settings using cells or tissues. It is important to note that these products are not categorized as medicines or drugs, and they have not received approval from the FDA for the prevention, treatment, or cure of any medical condition, ailment, or disease. We must emphasize that any form of bodily introduction of these products into humans or animals is strictly prohibited by law. It is essential to adhere to these guidelines to ensure compliance with legal and ethical standards in research and experimentation.