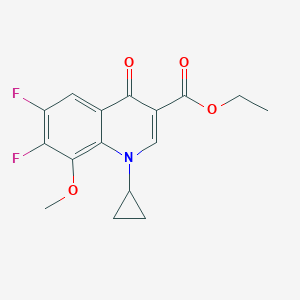
1-Cyclopropyl-6,7-difluoro-1,4-dihydro-8-methoxy-4-oxo-3-quinolinecarboxylic acid ethyl ester
Overview
Description
Ethyl 1-cyclopropyl-6,7-difluoro-8-methoxy-4-oxo-1,4-dihydroquinoline-3-carboxylate is a synthetic organic compound with the molecular formula C16H15F2NO4 It is a derivative of quinoline, a heterocyclic aromatic organic compound
Preparation Methods
Synthetic Routes and Reaction Conditions
The synthesis of ethyl 1-cyclopropyl-6,7-difluoro-8-methoxy-4-oxo-1,4-dihydroquinoline-3-carboxylate typically involves the cyclization of appropriate precursors under controlled conditions. One common method involves the reaction of ethyl α(Z)-[(diethylamino)methylene]-2,4,5-trifluoro-3-methoxy-β-oxo-benzenepropanoate with cyclopropylamine in a mixture of ethanol and diethyl ether . The reaction is carried out at room temperature, and the product is purified through recrystallization.
Industrial Production Methods
Industrial production of this compound may involve similar synthetic routes but on a larger scale. The process would be optimized for yield and purity, often involving automated systems for precise control of reaction conditions. The use of high-performance liquid chromatography (HPLC) and other analytical techniques ensures the quality of the final product.
Chemical Reactions Analysis
Types of Reactions
Ethyl 1-cyclopropyl-6,7-difluoro-8-methoxy-4-oxo-1,4-dihydroquinoline-3-carboxylate undergoes various chemical reactions, including:
Oxidation: This reaction can introduce additional functional groups or modify existing ones.
Reduction: This reaction can reduce the quinoline ring or other functional groups.
Substitution: This reaction can replace one functional group with another, often using nucleophilic or electrophilic reagents.
Common Reagents and Conditions
Oxidation: Common oxidizing agents include potassium permanganate (KMnO4) and chromium trioxide (CrO3).
Reduction: Common reducing agents include sodium borohydride (NaBH4) and lithium aluminum hydride (LiAlH4).
Substitution: Common reagents include halogens (e.g., chlorine, bromine) and organometallic compounds (e.g., Grignard reagents).
Major Products
The major products formed from these reactions depend on the specific conditions and reagents used. For example, oxidation may yield quinoline derivatives with additional oxygen-containing functional groups, while reduction may yield more saturated compounds.
Scientific Research Applications
Ethyl 1-cyclopropyl-6,7-difluoro-8-methoxy-4-oxo-1,4-dihydroquinoline-3-carboxylate has several scientific research applications:
Chemistry: It is used as a building block for the synthesis of more complex molecules.
Biology: It is studied for its potential biological activities, including antibacterial and antifungal properties.
Medicine: It is investigated for its potential use in developing new antibacterial agents, particularly against resistant strains of bacteria.
Industry: It is used in the production of pharmaceuticals and other fine chemicals.
Mechanism of Action
The mechanism of action of ethyl 1-cyclopropyl-6,7-difluoro-8-methoxy-4-oxo-1,4-dihydroquinoline-3-carboxylate involves its interaction with bacterial enzymes and DNA. It inhibits the activity of DNA gyrase and topoisomerase IV, enzymes essential for bacterial DNA replication and transcription. This inhibition leads to the disruption of bacterial cell division and ultimately cell death.
Comparison with Similar Compounds
Ethyl 1-cyclopropyl-6,7-difluoro-8-methoxy-4-oxo-1,4-dihydroquinoline-3-carboxylate is similar to other quinoline derivatives, such as:
Gatifloxacin: Another quinoline derivative with potent antibacterial activity.
Moxifloxacin: A fluoroquinolone antibiotic with a broad spectrum of activity.
Ciprofloxacin: A widely used fluoroquinolone antibiotic.
Uniqueness
What sets ethyl 1-cyclopropyl-6,7-difluoro-8-methoxy-4-oxo-1,4-dihydroquinoline-3-carboxylate apart is its specific structural modifications, which may confer unique antibacterial properties and potentially lower resistance development compared to other quinolines.
Properties
IUPAC Name |
ethyl 1-cyclopropyl-6,7-difluoro-8-methoxy-4-oxoquinoline-3-carboxylate | |
---|---|---|
Source | PubChem | |
URL | https://pubchem.ncbi.nlm.nih.gov | |
Description | Data deposited in or computed by PubChem | |
InChI |
InChI=1S/C16H15F2NO4/c1-3-23-16(21)10-7-19(8-4-5-8)13-9(14(10)20)6-11(17)12(18)15(13)22-2/h6-8H,3-5H2,1-2H3 | |
Source | PubChem | |
URL | https://pubchem.ncbi.nlm.nih.gov | |
Description | Data deposited in or computed by PubChem | |
InChI Key |
XPAOPAPDCRLMTR-UHFFFAOYSA-N | |
Source | PubChem | |
URL | https://pubchem.ncbi.nlm.nih.gov | |
Description | Data deposited in or computed by PubChem | |
Canonical SMILES |
CCOC(=O)C1=CN(C2=C(C(=C(C=C2C1=O)F)F)OC)C3CC3 | |
Source | PubChem | |
URL | https://pubchem.ncbi.nlm.nih.gov | |
Description | Data deposited in or computed by PubChem | |
Molecular Formula |
C16H15F2NO4 | |
Source | PubChem | |
URL | https://pubchem.ncbi.nlm.nih.gov | |
Description | Data deposited in or computed by PubChem | |
Molecular Weight |
323.29 g/mol | |
Source | PubChem | |
URL | https://pubchem.ncbi.nlm.nih.gov | |
Description | Data deposited in or computed by PubChem | |
CAS No. |
112811-71-9 | |
Record name | Moxifloxacin difluoro methoxy ethyl ester | |
Source | ChemIDplus | |
URL | https://pubchem.ncbi.nlm.nih.gov/substance/?source=chemidplus&sourceid=0112811719 | |
Description | ChemIDplus is a free, web search system that provides access to the structure and nomenclature authority files used for the identification of chemical substances cited in National Library of Medicine (NLM) databases, including the TOXNET system. | |
Record name | Difluormethoxychinoloncarbonsäureethylester | |
Source | European Chemicals Agency (ECHA) | |
URL | https://echa.europa.eu/information-on-chemicals | |
Description | The European Chemicals Agency (ECHA) is an agency of the European Union which is the driving force among regulatory authorities in implementing the EU's groundbreaking chemicals legislation for the benefit of human health and the environment as well as for innovation and competitiveness. | |
Explanation | Use of the information, documents and data from the ECHA website is subject to the terms and conditions of this Legal Notice, and subject to other binding limitations provided for under applicable law, the information, documents and data made available on the ECHA website may be reproduced, distributed and/or used, totally or in part, for non-commercial purposes provided that ECHA is acknowledged as the source: "Source: European Chemicals Agency, http://echa.europa.eu/". Such acknowledgement must be included in each copy of the material. ECHA permits and encourages organisations and individuals to create links to the ECHA website under the following cumulative conditions: Links can only be made to webpages that provide a link to the Legal Notice page. | |
Record name | MOXIFLOXACIN DIFLUORO METHOXY ETHYL ESTER | |
Source | FDA Global Substance Registration System (GSRS) | |
URL | https://gsrs.ncats.nih.gov/ginas/app/beta/substances/X3CH2A9DUL | |
Description | The FDA Global Substance Registration System (GSRS) enables the efficient and accurate exchange of information on what substances are in regulated products. Instead of relying on names, which vary across regulatory domains, countries, and regions, the GSRS knowledge base makes it possible for substances to be defined by standardized, scientific descriptions. | |
Explanation | Unless otherwise noted, the contents of the FDA website (www.fda.gov), both text and graphics, are not copyrighted. They are in the public domain and may be republished, reprinted and otherwise used freely by anyone without the need to obtain permission from FDA. Credit to the U.S. Food and Drug Administration as the source is appreciated but not required. | |
Synthesis routes and methods I
Procedure details
Synthesis routes and methods II
Procedure details
Retrosynthesis Analysis
AI-Powered Synthesis Planning: Our tool employs the Template_relevance Pistachio, Template_relevance Bkms_metabolic, Template_relevance Pistachio_ringbreaker, Template_relevance Reaxys, Template_relevance Reaxys_biocatalysis model, leveraging a vast database of chemical reactions to predict feasible synthetic routes.
One-Step Synthesis Focus: Specifically designed for one-step synthesis, it provides concise and direct routes for your target compounds, streamlining the synthesis process.
Accurate Predictions: Utilizing the extensive PISTACHIO, BKMS_METABOLIC, PISTACHIO_RINGBREAKER, REAXYS, REAXYS_BIOCATALYSIS database, our tool offers high-accuracy predictions, reflecting the latest in chemical research and data.
Strategy Settings
Precursor scoring | Relevance Heuristic |
---|---|
Min. plausibility | 0.01 |
Model | Template_relevance |
Template Set | Pistachio/Bkms_metabolic/Pistachio_ringbreaker/Reaxys/Reaxys_biocatalysis |
Top-N result to add to graph | 6 |
Feasible Synthetic Routes
Q1: What is the significance of Ethyl 1-cyclopropyl-6,7-difluoro-8-methoxy-4-oxo-1,4-dihydroquinoline-3-carboxylate in medicinal chemistry?
A1: Ethyl 1-cyclopropyl-6,7-difluoro-8-methoxy-4-oxo-1,4-dihydroquinoline-3-carboxylate serves as a crucial starting material for synthesizing new fluoroquinolone derivatives. Fluoroquinolones are a class of synthetic antibacterial agents known for their broad spectrum of activity. This specific compound provides a scaffold that can be chemically modified to potentially enhance antibacterial activity or target specific bacterial strains. [, ]
Q2: How is Ethyl 1-cyclopropyl-6,7-difluoro-8-methoxy-4-oxo-1,4-dihydroquinoline-3-carboxylate typically used in chemical synthesis?
A2: This compound is frequently utilized as a building block in reactions involving the modification of its carboxylate group. For example, it can be reacted with various amines in the presence of suitable reagents to yield substituted fluoroquinolone analogs. This approach allows for the introduction of diverse chemical moieties, potentially influencing the pharmacological profile of the resulting compounds. []
Q3: Are there any advantages to using microwave irradiation in the synthesis of fluoroquinolone analogs from Ethyl 1-cyclopropyl-6,7-difluoro-8-methoxy-4-oxo-1,4-dihydroquinoline-3-carboxylate?
A3: Research suggests that employing microwave irradiation in the synthesis of these compounds offers several benefits compared to conventional heating methods. These advantages include reduced reaction times, improved yields, and greater selectivity for desired products. [] This highlights the potential for microwave-assisted synthesis to enhance the efficiency of fluoroquinolone analog production.
Disclaimer and Information on In-Vitro Research Products
Please be aware that all articles and product information presented on BenchChem are intended solely for informational purposes. The products available for purchase on BenchChem are specifically designed for in-vitro studies, which are conducted outside of living organisms. In-vitro studies, derived from the Latin term "in glass," involve experiments performed in controlled laboratory settings using cells or tissues. It is important to note that these products are not categorized as medicines or drugs, and they have not received approval from the FDA for the prevention, treatment, or cure of any medical condition, ailment, or disease. We must emphasize that any form of bodily introduction of these products into humans or animals is strictly prohibited by law. It is essential to adhere to these guidelines to ensure compliance with legal and ethical standards in research and experimentation.