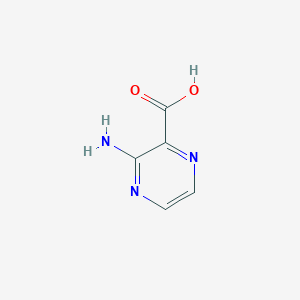
3-Aminopyrazine-2-carboxylic acid
Overview
Description
3-Aminopyrazine-2-carboxylic acid is an organic compound with the molecular formula C5H5N3O2 It is a derivative of pyrazine, characterized by the presence of an amino group at the third position and a carboxylic acid group at the second position on the pyrazine ring
Mechanism of Action
Target of Action
3-Aminopyrazine-2-carboxylic acid (3-APC) is a versatile compound with a wide range of biological activities. It has been reported to have significant antimicrobial activity . The primary targets of 3-APC are the microbial cells, where it interacts with various cellular components to exert its antimicrobial effects .
Mode of Action
It is known that the compound interacts with its targets in the microbial cells, leading to changes that inhibit the growth and proliferation of the microbes . The presence of the amino group in position 3 on the pyrazine ring is thought to play a crucial role in this interaction .
Biochemical Pathways
It is known that the compound interferes with the normal functioning of the microbial cells, leading to their death . The exact pathways and downstream effects are subject to ongoing research.
Result of Action
The result of the action of 3-APC is the inhibition of the growth and proliferation of microbial cells . This leads to a decrease in the number of microbes, thereby exerting its antimicrobial effects .
Preparation Methods
Synthetic Routes and Reaction Conditions
3-Aminopyrazine-2-carboxylic acid can be synthesized through several methods. One common approach involves the reaction of 2-amino-4-hydroxypteridine with alkali metal hydroxides at elevated temperatures (140-220°C). The product is then precipitated as a carboxylic acid by acidification .
Another method involves the synthesis from diethyl malonate, which undergoes a series of reactions including hydrogenation and bromination, to yield this compound .
Industrial Production Methods
Industrial production of this compound often employs scalable synthetic routes that are economical and practical. For instance, the synthesis of favipiravir, an antiviral drug, uses this compound as a key intermediate. The process involves multiple steps, including fluorination, hydroxylation, and nitrile hydrolysis, to achieve high yields and purity without the need for chromatographic purification .
Chemical Reactions Analysis
Types of Reactions
3-Aminopyrazine-2-carboxylic acid undergoes various chemical reactions, including:
Oxidation: It can be oxidized to form corresponding oxides.
Reduction: Reduction reactions can yield amine derivatives.
Substitution: The amino and carboxylic acid groups can participate in substitution reactions to form various derivatives.
Common Reagents and Conditions
Common reagents used in these reactions include alkali metal hydroxides for synthesis, hydrogenation catalysts for reduction, and various organic reagents for substitution reactions. Reaction conditions typically involve elevated temperatures and controlled pH levels to ensure the desired transformations.
Major Products
Major products formed from these reactions include various substituted pyrazine derivatives, which can be further utilized in the synthesis of complex organic molecules and pharmaceutical compounds .
Scientific Research Applications
3-Aminopyrazine-2-carboxylic acid has a wide range of applications in scientific research:
Comparison with Similar Compounds
3-Aminopyrazine-2-carboxylic acid can be compared with other similar compounds, such as:
Pyrazinamide: Another pyrazine derivative used as an antitubercular agent.
2-Aminopyrazine: A simpler derivative with applications in organic synthesis.
3-Hydroxypyrazine-2-carboxylic acid: A related compound with different functional groups and properties.
The uniqueness of this compound lies in its specific functional groups, which confer distinct chemical reactivity and biological activity, making it a versatile compound for various applications.
Properties
IUPAC Name |
3-aminopyrazine-2-carboxylic acid | |
---|---|---|
Source | PubChem | |
URL | https://pubchem.ncbi.nlm.nih.gov | |
Description | Data deposited in or computed by PubChem | |
InChI |
InChI=1S/C5H5N3O2/c6-4-3(5(9)10)7-1-2-8-4/h1-2H,(H2,6,8)(H,9,10) | |
Source | PubChem | |
URL | https://pubchem.ncbi.nlm.nih.gov | |
Description | Data deposited in or computed by PubChem | |
InChI Key |
ZAGZIOYVEIDDJA-UHFFFAOYSA-N | |
Source | PubChem | |
URL | https://pubchem.ncbi.nlm.nih.gov | |
Description | Data deposited in or computed by PubChem | |
Canonical SMILES |
C1=CN=C(C(=N1)C(=O)O)N | |
Source | PubChem | |
URL | https://pubchem.ncbi.nlm.nih.gov | |
Description | Data deposited in or computed by PubChem | |
Molecular Formula |
C5H5N3O2 | |
Source | PubChem | |
URL | https://pubchem.ncbi.nlm.nih.gov | |
Description | Data deposited in or computed by PubChem | |
DSSTOX Substance ID |
DTXSID20202589 | |
Record name | 3-Aminopyrazine-2-carboxylic acid | |
Source | EPA DSSTox | |
URL | https://comptox.epa.gov/dashboard/DTXSID20202589 | |
Description | DSSTox provides a high quality public chemistry resource for supporting improved predictive toxicology. | |
Molecular Weight |
139.11 g/mol | |
Source | PubChem | |
URL | https://pubchem.ncbi.nlm.nih.gov | |
Description | Data deposited in or computed by PubChem | |
CAS No. |
5424-01-1 | |
Record name | 3-Amino-2-pyrazinecarboxylic acid | |
Source | CAS Common Chemistry | |
URL | https://commonchemistry.cas.org/detail?cas_rn=5424-01-1 | |
Description | CAS Common Chemistry is an open community resource for accessing chemical information. Nearly 500,000 chemical substances from CAS REGISTRY cover areas of community interest, including common and frequently regulated chemicals, and those relevant to high school and undergraduate chemistry classes. This chemical information, curated by our expert scientists, is provided in alignment with our mission as a division of the American Chemical Society. | |
Explanation | The data from CAS Common Chemistry is provided under a CC-BY-NC 4.0 license, unless otherwise stated. | |
Record name | 3-Aminopyrazine-2-carboxylic acid | |
Source | ChemIDplus | |
URL | https://pubchem.ncbi.nlm.nih.gov/substance/?source=chemidplus&sourceid=0005424011 | |
Description | ChemIDplus is a free, web search system that provides access to the structure and nomenclature authority files used for the identification of chemical substances cited in National Library of Medicine (NLM) databases, including the TOXNET system. | |
Record name | 5424-01-1 | |
Source | DTP/NCI | |
URL | https://dtp.cancer.gov/dtpstandard/servlet/dwindex?searchtype=NSC&outputformat=html&searchlist=225114 | |
Description | The NCI Development Therapeutics Program (DTP) provides services and resources to the academic and private-sector research communities worldwide to facilitate the discovery and development of new cancer therapeutic agents. | |
Explanation | Unless otherwise indicated, all text within NCI products is free of copyright and may be reused without our permission. Credit the National Cancer Institute as the source. | |
Record name | 5424-01-1 | |
Source | DTP/NCI | |
URL | https://dtp.cancer.gov/dtpstandard/servlet/dwindex?searchtype=NSC&outputformat=html&searchlist=13148 | |
Description | The NCI Development Therapeutics Program (DTP) provides services and resources to the academic and private-sector research communities worldwide to facilitate the discovery and development of new cancer therapeutic agents. | |
Explanation | Unless otherwise indicated, all text within NCI products is free of copyright and may be reused without our permission. Credit the National Cancer Institute as the source. | |
Record name | 3-Aminopyrazine-2-carboxylic acid | |
Source | EPA DSSTox | |
URL | https://comptox.epa.gov/dashboard/DTXSID20202589 | |
Description | DSSTox provides a high quality public chemistry resource for supporting improved predictive toxicology. | |
Record name | 3-aminopyrazine-2-carboxylic acid | |
Source | European Chemicals Agency (ECHA) | |
URL | https://echa.europa.eu/substance-information/-/substanceinfo/100.024.144 | |
Description | The European Chemicals Agency (ECHA) is an agency of the European Union which is the driving force among regulatory authorities in implementing the EU's groundbreaking chemicals legislation for the benefit of human health and the environment as well as for innovation and competitiveness. | |
Explanation | Use of the information, documents and data from the ECHA website is subject to the terms and conditions of this Legal Notice, and subject to other binding limitations provided for under applicable law, the information, documents and data made available on the ECHA website may be reproduced, distributed and/or used, totally or in part, for non-commercial purposes provided that ECHA is acknowledged as the source: "Source: European Chemicals Agency, http://echa.europa.eu/". Such acknowledgement must be included in each copy of the material. ECHA permits and encourages organisations and individuals to create links to the ECHA website under the following cumulative conditions: Links can only be made to webpages that provide a link to the Legal Notice page. | |
Record name | 2-AMINO-3-CARBOXYPYRAZINE | |
Source | FDA Global Substance Registration System (GSRS) | |
URL | https://gsrs.ncats.nih.gov/ginas/app/beta/substances/X78B5D12FN | |
Description | The FDA Global Substance Registration System (GSRS) enables the efficient and accurate exchange of information on what substances are in regulated products. Instead of relying on names, which vary across regulatory domains, countries, and regions, the GSRS knowledge base makes it possible for substances to be defined by standardized, scientific descriptions. | |
Explanation | Unless otherwise noted, the contents of the FDA website (www.fda.gov), both text and graphics, are not copyrighted. They are in the public domain and may be republished, reprinted and otherwise used freely by anyone without the need to obtain permission from FDA. Credit to the U.S. Food and Drug Administration as the source is appreciated but not required. | |
Retrosynthesis Analysis
AI-Powered Synthesis Planning: Our tool employs the Template_relevance Pistachio, Template_relevance Bkms_metabolic, Template_relevance Pistachio_ringbreaker, Template_relevance Reaxys, Template_relevance Reaxys_biocatalysis model, leveraging a vast database of chemical reactions to predict feasible synthetic routes.
One-Step Synthesis Focus: Specifically designed for one-step synthesis, it provides concise and direct routes for your target compounds, streamlining the synthesis process.
Accurate Predictions: Utilizing the extensive PISTACHIO, BKMS_METABOLIC, PISTACHIO_RINGBREAKER, REAXYS, REAXYS_BIOCATALYSIS database, our tool offers high-accuracy predictions, reflecting the latest in chemical research and data.
Strategy Settings
Precursor scoring | Relevance Heuristic |
---|---|
Min. plausibility | 0.01 |
Model | Template_relevance |
Template Set | Pistachio/Bkms_metabolic/Pistachio_ringbreaker/Reaxys/Reaxys_biocatalysis |
Top-N result to add to graph | 6 |
Feasible Synthetic Routes
Q1: What is the molecular formula and weight of 3-aminopyrazine-2-carboxylic acid?
A: The molecular formula of this compound is C5H5N3O2, and its molecular weight is 139.11 g/mol. [, ]
Q2: What spectroscopic data is available for this compound?
A: Studies have characterized this compound using various spectroscopic techniques, including infrared (IR) spectroscopy, 1H NMR, 13C NMR, and UV-Vis spectroscopy. These analyses provide insights into its structural features, functional groups, and electronic properties. [, , , ] Additionally, FTIR spectroscopy has been employed to study its isomerization upon UV irradiation in argon matrices. []
Q3: How does the structure of this compound influence its hydrogen bonding capabilities?
A: this compound exhibits an extensive network of intra- and intermolecular hydrogen bonds. This is attributed to the presence of both a carboxylic acid group and an amine group, which act as hydrogen bond donors and acceptors, respectively. []
Q4: Is this compound stable under various conditions?
A: Research suggests that this compound exhibits stability under a range of conditions. Studies have investigated its thermal stability using thermogravimetric analysis (TGA) in the context of coordination polymers. [, ] Furthermore, its stability in different solvents and under various pH levels has been explored for applications like complex synthesis and formulation development. [, ]
Q5: What types of metal complexes can be formed with this compound?
A: this compound (Hapca) readily forms complexes with various transition metals, lanthanides, and alkali metals. Researchers have synthesized and characterized complexes with VO2+, Pd(II), W(VI), UO22+, Cu(II), Ag(I), Zn(II), Mn(II), Co(II), Ni(II), Cd(II), Pb(II), various lanthanides, and Na+. [, , , , , , , , , , , , ]
Q6: What coordination modes does this compound exhibit in these complexes?
A6: The ligand can coordinate to metal centers through various modes, including:
- Bidentate chelation: Coordinating through the pyrazine nitrogen and one carboxylate oxygen atom. [, , , , ]
- Bridging modes: Utilizing one or both carboxylate oxygen atoms to bridge multiple metal centers. [, ]
Q7: What are the potential applications of this compound-based metal complexes?
A7: Research has explored the potential of these complexes in various fields, including:
- Luminescent materials: Lanthanide complexes exhibit interesting luminescent properties with potential applications in sensing, lighting, and display technologies. [, , ]
- Catalysis: Pb(II) complexes have demonstrated catalytic activity in cyanosilylation reactions, showcasing the potential of this compound-based complexes in organic synthesis. []
- Anticancer agents: Studies have investigated the anticancer activity of transition metal complexes with this compound. []
Q8: Have computational methods been used to study this compound and its complexes?
A: Yes, computational chemistry has been employed to investigate the molecular structure, bonding characteristics, and redox properties of this compound and its transition metal complexes. []
Q9: Are there any structure-activity relationship (SAR) studies available for this compound derivatives?
A: While limited SAR data exists specifically for this compound, research on its derivatives, particularly esters, highlights the influence of structural modifications on biological activity. For example, a study investigating the antimycobacterial activity of aminopyrazinoic acid esters identified hexyl 3-aminopyrazine-2-carboxylate as the most potent compound against Mycobacterium tuberculosis H37Rv. [] These findings emphasize the importance of the ester moiety and alkyl chain length for enhancing activity.
Q10: How is this compound synthesized?
A10: A common synthetic route starts from pyrazine-2,3-dicarboxylic acid and involves a three-step process:
- Hofmann rearrangement: The ammonium salt undergoes a Hofmann rearrangement to yield this compound. []
Q11: What analytical methods are used to characterize and quantify this compound?
A11: Common analytical techniques used include:
- Spectroscopic methods: IR, NMR (1H and 13C), UV-Vis, and FTIR spectroscopy are employed for structural elucidation and identification. [, , , , ]
- Elemental analysis: Determining the elemental composition of the compound to confirm its purity and identity. [, , , , ]
- X-ray crystallography: Used to determine the three-dimensional structure of the compound and its complexes in the solid state. [, , , , , , , , , , , , ]
- Thermogravimetric analysis (TGA): Assessing the thermal stability of the compound and its complexes. [, ]
Q12: What is the known biological activity of this compound and its derivatives?
A12: Research suggests potential biological activities for this compound and its derivatives, including:
- Antimycobacterial activity: Esters of this compound have shown promising in vitro activity against Mycobacterium tuberculosis. []
- Insulino-mimetic activity: Some vanadium and zinc complexes with this compound have demonstrated the ability to inhibit free fatty acid release from rat adipocytes, suggesting potential as insulin-sensitizing agents. []
Disclaimer and Information on In-Vitro Research Products
Please be aware that all articles and product information presented on BenchChem are intended solely for informational purposes. The products available for purchase on BenchChem are specifically designed for in-vitro studies, which are conducted outside of living organisms. In-vitro studies, derived from the Latin term "in glass," involve experiments performed in controlled laboratory settings using cells or tissues. It is important to note that these products are not categorized as medicines or drugs, and they have not received approval from the FDA for the prevention, treatment, or cure of any medical condition, ailment, or disease. We must emphasize that any form of bodily introduction of these products into humans or animals is strictly prohibited by law. It is essential to adhere to these guidelines to ensure compliance with legal and ethical standards in research and experimentation.