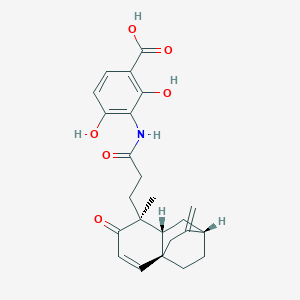
Platencin
Overview
Description
Platencin is a natural antibiotic compound produced by the bacterium Streptomyces platensis. It was discovered alongside platensimycin and has garnered significant attention due to its potent antibacterial properties. This compound is particularly effective against Gram-positive pathogens, including antibiotic-resistant strains such as methicillin-resistant Staphylococcus aureus and vancomycin-resistant enterococci .
Mechanism of Action
Target of Action
Platencin, a natural antibiotic produced by Streptomyces platensis, primarily targets two key enzymes in bacterial fatty acid synthesis: β-ketoacyl-ACP synthase II (FabF) and β-ketoacyl-ACP synthase III (FabH) . These enzymes play a crucial role in the elongation of the fatty acid chain, which is essential for the production of bacterial cell membranes .
Mode of Action
This compound inhibits the action of FabF and FabH enzymes, thereby halting the elongation of the fatty acid chain . This inhibition is achieved through specific conformational changes known as the ‘open configuration’, which allows this compound to bind to the active site of these enzymes . Once bound, this compound engages in hydrogen-bonding interactions with key amino acid residues, which is highly dependent upon the conformation of the cyclohexenone ring .
Biochemical Pathways
By inhibiting FabF and FabH, this compound disrupts the fatty acid biosynthesis pathway of type II . The disruption of this pathway leads to the inhibition of the production of fatty acids required for bacterial cell membranes .
Pharmacokinetics
While this compound shows potent antibacterial activity, its pharmacokinetics must be improved for it to become a successful drug . It has a high rate of clearance in the body, yielding a low degree of systematic exposure .
Result of Action
The result of this compound’s action is a broad-spectrum Gram-positive antibacterial activity . . This makes this compound a promising candidate for treating infections caused by these resistant strains.
Action Environment
The action, efficacy, and stability of this compound can be influenced by various environmental factors. For instance, the production of this compound can be significantly improved by optimizing the fermentation medium and engineering the biosynthetic pathway . Additionally, the use of rational metabolic engineering has led to the production of superior strains that produce hundreds of times more antibiotic than the natural strains .
Biochemical Analysis
Biochemical Properties
Platencin plays a significant role in biochemical reactions by inhibiting the enzymes β-ketoacyl-[acyl carrier protein (ACP)] synthase II and III (FabF and FabH) with IC50 values of 1.95 μg/ml and 3.91 μg/ml, respectively . These interactions disrupt the normal function of these enzymes, leading to its broad-spectrum Gram-positive antibacterial activity .
Cellular Effects
This compound exhibits a broad-spectrum Gram-positive antibacterial activity through inhibition of fatty acid biosynthesis . It does not exhibit cross-resistance to key antibiotic-resistant strains tested, including methicillin-resistant Staphylococcus aureus, vancomycin-intermediate S. aureus, and vancomycin-resistant Enterococci .
Molecular Mechanism
The molecular mechanism of this compound involves the inhibition of fatty acid biosynthesis. It targets two essential proteins, β-ketoacyl-[acyl carrier protein (ACP)] synthase II (FabF) and III (FabH), emphasizing the fact that more antibiotics with novel structures and new modes of action can be discovered .
Dosage Effects in Animal Models
Early studies suggest it shows potent in vivo efficacy without any observed toxicity .
Metabolic Pathways
This compound is involved in the metabolic pathway of fatty acid biosynthesis . It interacts with the enzymes FabF and FabH, disrupting their normal function and leading to its antibacterial activity .
Subcellular Localization
Given its role in inhibiting enzymes involved in fatty acid biosynthesis, it is likely to be found in areas of the cell where these processes occur .
Preparation Methods
Synthetic Routes and Reaction Conditions: The synthesis of platencin involves several key steps, including the intramolecular Diels-Alder reaction of masked o-benzoquinone, intramolecular aldol condensation, and stereoselective hydrogenation . A concise formal synthesis of this compound has been realized, featuring an organocatalytic approach to the [2.2.2] bicyclic core, a radical reductive elimination, a gold-catalyzed Meyer–Schuster rearrangement, and a rhodium-catalyzed chemo- and diastereoselective hydrosilylation .
Industrial Production Methods: Industrial production of this compound has been optimized through biosynthetic pathway engineering and fermentation medium optimization. The implementation of a CRISPR-Cas9 system in Streptomyces platensis has enabled the construction of recombinant strains for the overproduction of this compound .
Chemical Reactions Analysis
Types of Reactions: Platencin undergoes various chemical reactions, including oxidation, reduction, and substitution. The intramolecular Diels-Alder reaction and aldol condensation are key steps in its synthesis .
Common Reagents and Conditions:
Oxidation: Thallium (III) nitrate or hypervalent iodines in the presence of an appropriate alcohol.
Reduction: Stereoselective hydrogenation.
Substitution: Organocatalytic approaches and radical reductive elimination.
Major Products: The major products formed from these reactions include the tricyclic core structure of this compound and its derivatives .
Scientific Research Applications
Antibacterial Properties
Platencin exhibits strong antibacterial activity against a range of Gram-positive pathogens, including:
- Methicillin-resistant Staphylococcus aureus (MRSA)
- Vancomycin-resistant enterococci (VRE)
- Mycobacterium tuberculosis
Research indicates that this compound operates through a unique mechanism by inhibiting two key enzymes in fatty acid synthesis: FabF and FabH. This dual inhibition is crucial as it targets bacterial lipid biosynthesis, which is essential for cell membrane integrity and function .
Table 1: Comparison of Inhibitory Activity Against Key Enzymes
Compound | Target Enzyme | IC50 (μM) |
---|---|---|
This compound | FabF | 4.58 |
This compound | FabH | 9.17 |
Platensimycin | FabF | 0.29 |
Platensimycin | FabH | 247 |
This table highlights that while this compound is less effective against FabF compared to platensimycin, it shows superior activity against FabH, indicating its potential as a complementary therapeutic agent in combating resistant bacterial strains .
Pharmacological Insights
The pharmacokinetic properties of this compound present both challenges and opportunities. While it has shown promising in vitro efficacy and low toxicity profiles, further studies are needed to enhance its bioavailability and therapeutic index in clinical settings . The compound's structural characteristics—specifically its tetracyclic unit—contribute to its unique mechanism of action and potential for further chemical modification to improve efficacy .
Research and Development
Recent advancements in microbial genomics have facilitated deeper insights into the biosynthesis of this compound and related compounds. Researchers have employed CRISPR-Cas9 technology to engineer strains of S. platensis for enhanced production of this compound, paving the way for more sustainable and efficient manufacturing processes .
Future Perspectives
Given the rising threat of antibiotic resistance, the exploration of natural products like this compound is increasingly critical. Its unique mode of action not only offers a pathway for developing new antibiotics but also inspires further research into natural product chemistry and microbial metabolism .
Case Study: this compound Against MRSA
A notable case study involved testing this compound's efficacy against clinical isolates of MRSA. In vitro assays demonstrated that this compound inhibited MRSA growth at concentrations that were non-toxic to human cells. This finding supports the compound's potential as a therapeutic option for treating infections caused by resistant strains .
Comparison with Similar Compounds
Platensimycin: Another antibiotic produced by Streptomyces platensis, which inhibits the elongation-condensing enzyme FabF.
Platensilin: A related compound with similar antibacterial properties.
Uniqueness of Platencin: this compound is unique in its dual inhibition of both FabF and FabH, whereas platensimycin selectively inhibits FabF . This dual inhibition makes this compound a more potent antibiotic against a broader range of bacterial pathogens.
Biological Activity
Platencin is a natural antibiotic compound derived from the bacterium Streptomyces platensis. It has garnered significant attention due to its unique mechanism of action and potent antibacterial properties, particularly against Gram-positive bacteria, including drug-resistant strains. This article delves into the biological activity of this compound, exploring its mechanisms, efficacy, and relevant research findings.
This compound was discovered through a high-throughput screening of over 250,000 natural product extracts aimed at identifying inhibitors of the fatty acid biosynthesis pathway in bacteria. It functions primarily as a dual inhibitor of two key enzymes in this pathway: FabF and FabH , which are essential for lipid elongation and initiation, respectively .
Chemical Structure
The chemical structure of this compound features a unique ketolide portion that distinguishes it from its congener platensimycin. Both compounds share a similar structural motif but differ in their hydrophobic characteristics, influencing their biological activity .
Antibacterial Efficacy
This compound exhibits broad-spectrum antibacterial activity with minimum inhibitory concentrations (MICs) reported as follows:
Bacterial Strain | MIC (μg/mL) |
---|---|
Methicillin-resistant Staphylococcus aureus (MRSA) | 1.0 |
Vancomycin-resistant Enterococcus faecium (VREF) | <0.06 |
Streptococcus pneumoniae | 4.0 |
These values indicate that this compound is particularly effective against resistant strains, making it a promising candidate for further development in antibiotic therapies .
In Vivo Studies
In vivo efficacy studies using mouse models have demonstrated that this compound effectively reduces bacterial load without significant toxicity. Continuous infusion models showed that it can effectively manage infections caused by S. aureus with no observable adverse effects on the host .
Comparative Studies with Platensimycin
While both this compound and platensimycin target the fatty acid biosynthesis pathway, their inhibitory potencies differ significantly:
Compound | FabF IC50 (μM) | FabH IC50 (μM) |
---|---|---|
This compound | 1.95 | 3.91 |
Platensimycin | 0.048 | 67 |
The data suggests that while platensimycin is a more potent inhibitor of FabF, this compound's dual inhibition strategy may confer advantages in terms of reducing the potential for bacterial resistance .
Resistance Mechanisms
Research indicates that mutations in the fabH gene can confer resistance to antibiotics targeting FabF and FabH, highlighting the need for ongoing studies to understand resistance mechanisms better . The dual-target approach of this compound may mitigate this risk by increasing the likelihood that at least one target remains susceptible.
Properties
CAS No. |
869898-86-2 |
---|---|
Molecular Formula |
C24H27NO6 |
Molecular Weight |
425.5 g/mol |
IUPAC Name |
2,4-dihydroxy-3-[3-[(1S,5S,8S)-5-methyl-9-methylidene-4-oxo-5-tricyclo[6.2.2.01,6]dodec-2-enyl]propanoylamino]benzoic acid |
InChI |
InChI=1S/C24H27NO6/c1-13-12-24-9-5-14(13)11-17(24)23(2,18(27)6-10-24)8-7-19(28)25-20-16(26)4-3-15(21(20)29)22(30)31/h3-4,6,10,14,17,26,29H,1,5,7-9,11-12H2,2H3,(H,25,28)(H,30,31)/t14-,17?,23-,24+/m0/s1 |
InChI Key |
DWUHGPPFFABTIY-KMVHLTCXSA-N |
SMILES |
CC1(C2CC3CCC2(CC3=C)C=CC1=O)CCC(=O)NC4=C(C=CC(=C4O)C(=O)O)O |
Isomeric SMILES |
C[C@@]1(C2C[C@@H]3CC[C@]2(CC3=C)C=CC1=O)CCC(=O)NC4=C(C=CC(=C4O)C(=O)O)O |
Canonical SMILES |
CC1(C2CC3CCC2(CC3=C)C=CC1=O)CCC(=O)NC4=C(C=CC(=C4O)C(=O)O)O |
Appearance |
Light tan solid |
Synonyms |
3-[[3-[(2S,8S)-1,3,4,7,8,8aR-hexahydro-8-methyl-3-methylene-7-oxo-2H-2,4aS-ethanon-aphthalen-8-yl]-1-oxopropyl]amino]-2,4-dihydroxy-benzoic acid |
Origin of Product |
United States |
Retrosynthesis Analysis
AI-Powered Synthesis Planning: Our tool employs the Template_relevance Pistachio, Template_relevance Bkms_metabolic, Template_relevance Pistachio_ringbreaker, Template_relevance Reaxys, Template_relevance Reaxys_biocatalysis model, leveraging a vast database of chemical reactions to predict feasible synthetic routes.
One-Step Synthesis Focus: Specifically designed for one-step synthesis, it provides concise and direct routes for your target compounds, streamlining the synthesis process.
Accurate Predictions: Utilizing the extensive PISTACHIO, BKMS_METABOLIC, PISTACHIO_RINGBREAKER, REAXYS, REAXYS_BIOCATALYSIS database, our tool offers high-accuracy predictions, reflecting the latest in chemical research and data.
Strategy Settings
Precursor scoring | Relevance Heuristic |
---|---|
Min. plausibility | 0.01 |
Model | Template_relevance |
Template Set | Pistachio/Bkms_metabolic/Pistachio_ringbreaker/Reaxys/Reaxys_biocatalysis |
Top-N result to add to graph | 6 |
Feasible Synthetic Routes
Disclaimer and Information on In-Vitro Research Products
Please be aware that all articles and product information presented on BenchChem are intended solely for informational purposes. The products available for purchase on BenchChem are specifically designed for in-vitro studies, which are conducted outside of living organisms. In-vitro studies, derived from the Latin term "in glass," involve experiments performed in controlled laboratory settings using cells or tissues. It is important to note that these products are not categorized as medicines or drugs, and they have not received approval from the FDA for the prevention, treatment, or cure of any medical condition, ailment, or disease. We must emphasize that any form of bodily introduction of these products into humans or animals is strictly prohibited by law. It is essential to adhere to these guidelines to ensure compliance with legal and ethical standards in research and experimentation.