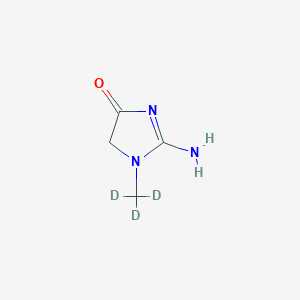
2-amino-3-(trideuteriomethyl)-4H-imidazol-5-one
Overview
Description
Creatinine-d3 is a stable isotope-labeled compound used primarily as an internal standard in mass spectrometry for the quantification of creatinine. It is synthesized in the kidney, liver, and pancreas and transported in the blood to muscle and brain, where it is phosphorylated to phosphocreatine . Creatinine-d3 is particularly valuable in clinical diagnostics, forensic analysis, and research applications due to its stability and accuracy in measurement.
Mechanism of Action
Target of Action
The primary target of 2-amino-3-(trideuteriomethyl)-4H-imidazol-5-one is the enzyme 2-amino-3-ketobutyrate coenzyme A ligase . This enzyme plays a crucial role in the metabolism of amino acids, particularly in the catabolic pathway of branched-chain amino acids .
Mode of Action
The compound interacts with its target enzyme, 2-amino-3-ketobutyrate coenzyme A ligase, and participates in the conversion of 2-amino-3-ketobutyrate to glycine and acetyl-CoA . This interaction results in changes in the metabolic pathways involving these molecules.
Biochemical Pathways
The compound affects the biochemical pathways involving the metabolism of branched-chain amino acids. Specifically, it is involved in the conversion of 2-amino-3-ketobutyrate to glycine and acetyl-CoA, a key step in the catabolic pathway of these amino acids . The downstream effects include the production of energy and other metabolic intermediates.
Pharmacokinetics
Similar compounds have been shown to have rapid distribution phases followed by slower elimination phases . The compound’s ADME properties and their impact on bioavailability would need further investigation.
Result of Action
The result of the compound’s action is the production of glycine and acetyl-CoA from 2-amino-3-ketobutyrate . This contributes to various metabolic processes, including energy production and the synthesis of other important molecules.
Action Environment
The action of this compound can be influenced by various environmental factors. For instance, the pH and temperature of the environment can affect the activity of the target enzyme and thus the efficacy of the compound. Additionally, the presence of other molecules can also influence the compound’s stability and action .
Biochemical Analysis
Biochemical Properties
2-amino-3-(trideuteriomethyl)-4H-imidazol-5-one plays a significant role in biochemical reactions, particularly in the context of enzyme interactions. This compound interacts with various enzymes, including threonine aldolases, which are pyridoxal-5-phosphate-dependent enzymes. Threonine aldolases catalyze the cleavage of threonine to glycine and acetaldehyde, and they also facilitate the reverse aldol reaction, forming chiral β-hydroxy-α-amino acids . The interaction between this compound and threonine aldolases is crucial for the synthesis of these chiral compounds, which are important precursors for pharmaceuticals.
Cellular Effects
This compound has notable effects on various types of cells and cellular processes. It influences cell function by modulating cell signaling pathways, gene expression, and cellular metabolism. For instance, the overexpression of genes involved in the kynurenine pathway, such as 2-amino-3-carboxymuconate-6-semialdehyde decarboxylase, can affect the expression levels of indoleamine 2,3-dioxygenase 1 and interferon-gamma in inflammatory conditions . This modulation of gene expression and signaling pathways highlights the potential impact of this compound on cellular processes.
Molecular Mechanism
The molecular mechanism of this compound involves its interaction with specific biomolecules, leading to enzyme inhibition or activation and changes in gene expression. For example, the condensation process catalyzed by 2-amino-3-oxobutyrate CoA ligase involves the loss of the pro-R hydrogen atom of glycine and occurs with the inversion of stereochemistry . This interaction is indicative of the compound’s ability to influence enzymatic reactions at the molecular level.
Temporal Effects in Laboratory Settings
In laboratory settings, the effects of this compound can change over time. Studies on similar compounds have shown that factors such as solvent polarity, pH, and the presence of β-cyclodextrin can influence the stability and degradation of the compound . These temporal effects are important for understanding the long-term impact of this compound on cellular function in both in vitro and in vivo studies.
Dosage Effects in Animal Models
The effects of this compound vary with different dosages in animal models. Research on related compounds, such as 2-amino-3-methylimidazo[4,5-f]quinoline, has demonstrated dose-dependent increases in hepatotoxicity and oxidative stress in zebrafish . These findings suggest that this compound may exhibit similar dosage effects, including potential toxic or adverse effects at high doses.
Metabolic Pathways
This compound is involved in various metabolic pathways, interacting with enzymes and cofactors that influence metabolic flux and metabolite levels. For instance, the degradation of aromatic hydrocarbons by bacteria involves upper and lower pathways, with enzymes catalyzing the conversion of the original compound to central intermediates and subsequent cleavage to yield metabolites for biomass production . Understanding these metabolic pathways is essential for elucidating the role of this compound in cellular metabolism.
Transport and Distribution
The transport and distribution of this compound within cells and tissues involve specific transporters and binding proteins. The L-type amino acid transporter 1 (LAT1) is one such transporter that mediates the exchange of essential amino acids across biological barriers . This transporter is predominantly expressed in various tissues, including the cerebral cortex, blood-brain barrier, and several types of cancer, facilitating the targeted delivery of compounds like this compound.
Subcellular Localization
The subcellular localization of this compound is influenced by targeting signals and post-translational modifications that direct it to specific compartments or organelles. For example, the inclusion complex formation with β-cyclodextrin can affect the localization and activity of the compound within cells . Understanding the subcellular localization is crucial for determining the functional role of this compound in cellular processes.
Preparation Methods
Synthetic Routes and Reaction Conditions
The synthesis of Creatinine-d3 involves the incorporation of deuterium atoms into the creatinine molecule. This is typically achieved through a series of chemical reactions that replace hydrogen atoms with deuterium. The process often starts with the synthesis of deuterated precursors, followed by their conversion into Creatinine-d3 through specific reaction conditions .
Industrial Production Methods
Industrial production of Creatinine-d3 involves large-scale chemical synthesis using deuterated reagents. The process is optimized for high yield and purity, ensuring that the final product meets the stringent requirements for use as an internal standard in analytical applications .
Chemical Reactions Analysis
Types of Reactions
Creatinine-d3 undergoes various chemical reactions, including:
Oxidation: Creatinine-d3 can be oxidized to form creatine.
Reduction: It can be reduced back to creatine under specific conditions.
Substitution: Deuterium atoms in Creatinine-d3 can be replaced with hydrogen atoms in certain reactions.
Common Reagents and Conditions
Common reagents used in these reactions include oxidizing agents like potassium permanganate and reducing agents like sodium borohydride. The reactions are typically carried out under controlled conditions to ensure the desired product is formed .
Major Products
The major products formed from these reactions include creatine and other deuterated derivatives, which are used in various analytical and research applications .
Scientific Research Applications
Creatinine-d3 has a wide range of scientific research applications, including:
Comparison with Similar Compounds
Similar Compounds
Creatinine: The non-deuterated form of Creatinine-d3, used in similar applications but without the isotope labeling.
Creatine: A precursor to creatinine, involved in energy metabolism in muscle.
Phosphocreatine: A phosphorylated form of creatine, serving as an energy reservoir in muscle cells.
Uniqueness
Creatinine-d3 is unique due to its stable isotope labeling, which provides enhanced accuracy and reliability in analytical measurements. This makes it particularly valuable in clinical diagnostics and research applications where precise quantification is essential .
Properties
CAS No. |
143827-20-7 |
---|---|
Molecular Formula |
C4H7N3O |
Molecular Weight |
116.14 g/mol |
IUPAC Name |
2-imino-1-(trideuteriomethyl)imidazolidin-4-one |
InChI |
InChI=1S/C4H7N3O/c1-7-2-3(8)6-4(7)5/h2H2,1H3,(H2,5,6,8)/i1D3 |
InChI Key |
DDRJAANPRJIHGJ-FIBGUPNXSA-N |
Isomeric SMILES |
[2H]C([2H])([2H])N1CC(=O)NC1=N |
SMILES |
CN1CC(=O)N=C1N |
Canonical SMILES |
CN1CC(=O)NC1=N |
Appearance |
Assay:≥98% deuterated forms (d1-d3)A crystalline solid |
Synonyms |
2-Amino-1,5-dihydro-1-(methyl-d3)-4H-imidazol-4-one; 1-(Methyl-d3)glycocyamidine; 1-(Methyl-d3)hydantoin-2-imide; 2-Amino-1-(methyl-d3)-1,5-dihydroimidazol-4-one; 2-Amino-1-(methyl-d3)imidazolin-4-one; NSC 13123-d3; TEGO Cosmo C 250-d3; |
Origin of Product |
United States |
Retrosynthesis Analysis
AI-Powered Synthesis Planning: Our tool employs the Template_relevance Pistachio, Template_relevance Bkms_metabolic, Template_relevance Pistachio_ringbreaker, Template_relevance Reaxys, Template_relevance Reaxys_biocatalysis model, leveraging a vast database of chemical reactions to predict feasible synthetic routes.
One-Step Synthesis Focus: Specifically designed for one-step synthesis, it provides concise and direct routes for your target compounds, streamlining the synthesis process.
Accurate Predictions: Utilizing the extensive PISTACHIO, BKMS_METABOLIC, PISTACHIO_RINGBREAKER, REAXYS, REAXYS_BIOCATALYSIS database, our tool offers high-accuracy predictions, reflecting the latest in chemical research and data.
Strategy Settings
Precursor scoring | Relevance Heuristic |
---|---|
Min. plausibility | 0.01 |
Model | Template_relevance |
Template Set | Pistachio/Bkms_metabolic/Pistachio_ringbreaker/Reaxys/Reaxys_biocatalysis |
Top-N result to add to graph | 6 |
Feasible Synthetic Routes
Disclaimer and Information on In-Vitro Research Products
Please be aware that all articles and product information presented on BenchChem are intended solely for informational purposes. The products available for purchase on BenchChem are specifically designed for in-vitro studies, which are conducted outside of living organisms. In-vitro studies, derived from the Latin term "in glass," involve experiments performed in controlled laboratory settings using cells or tissues. It is important to note that these products are not categorized as medicines or drugs, and they have not received approval from the FDA for the prevention, treatment, or cure of any medical condition, ailment, or disease. We must emphasize that any form of bodily introduction of these products into humans or animals is strictly prohibited by law. It is essential to adhere to these guidelines to ensure compliance with legal and ethical standards in research and experimentation.