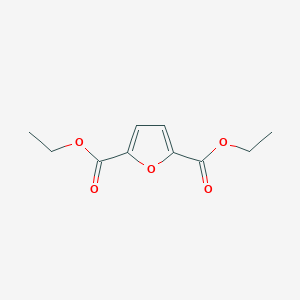
Diethyl furan-2,5-dicarboxylate
Overview
Description
Diethyl furan-2,5-dicarboxylate is a structural analog of diacids . It is a compound with the molecular formula C10H12O5 .
Synthesis Analysis
Diethyl furan-2,5-dicarboxylate has been synthesized by the reaction of 5-hydroxymethylfurfural with ethyl esters and phosphotungstic acid . It has also been used in the lipase-catalyzed synthesis of semiaromatic copolyesters . A stepwise approach involving a protecting agent has been used to obtain dimethyl furan-2,5-dicarboxylate, a monomer for polyalkylene furanoates .Molecular Structure Analysis
The molecular structure of Diethyl furan-2,5-dicarboxylate has been determined using various techniques such as Fourier transform infrared spectroscopy and nuclear magnetic resonance . The InChI string for this compound is InChI=1S/C10H12O5/c1-3-13-9(11)7-5-6-8(15-7)10(12)14-4-2/h5-6H,3-4H2,1-2H3 .Chemical Reactions Analysis
The reaction of Diethyl furan-2,5-dicarboxylate proceeds through direct esterification or transesterification of FDCA or its corresponding ester, with polyhydroxy alcohol in the presence of a catalyst with an excess of diols to obtain oligomers, followed by the polycondensation reaction .Physical And Chemical Properties Analysis
Diethyl furan-2,5-dicarboxylate has a molecular weight of 212.20 g/mol . It has a topological polar surface area of 65.7 Ų and a complexity of 214 .Scientific Research Applications
Production of Furanic Polyesters
Diethyl furan-2,5-dicarboxylate (DEFDC) is used in the synthesis of furanic polyesters. A one-pot two-step procedure has been developed for the synthesis of DEFDC starting from mucic acid . This process involves the production of three different kinds of furan-based polyesters— polyethylene-2,5-furan dicarboxylate (PEF), polyhydropropyl-2,5-furan dicarboxylate (PHPF), and polydiglycerol-2,5-furandicarboxylate (PDGF) .
Oxidative Esterification
DEFDC can be prepared through a one-step oxidative esterification of 2,5-furandiformaldehyde (DFF) derived from biomass .
Synthesis of Biobased Polymers
DEFDC is used in the synthesis of biobased polymers . These polymers are characterized by NMR, FT-IR spectroscopy, DSC, TGA, and XRD .
Production of Polyethylene 2,5-furandicarboxylate (PEF)
DEFDC is used in the production of Polyethylene 2,5-furandicarboxylate (PEF), a promising biomass molecule for polymerization with ethylene glycol . PEF can be used as an alternative material to polyethylene terephthalate (PET) .
Synthesis of Pyrrolo[1,2-b]pyridazines
Although not directly related to DEFDC, it’s worth noting that similar compounds such as Diethyl pyrrole-2,5-dicarboxylate have found uses in medicinal chemistry as a precursor in the synthesis of pyrrolo[1,2-b]pyridazines for the treatment of proliferative disorders .
Potential Janus Kinase Inhibitors
Again, similar compounds like Diethyl pyrrole-2,5-dicarboxylate have been used in the development of potential Janus kinase inhibitors .
Safety And Hazards
Future Directions
properties
IUPAC Name |
diethyl furan-2,5-dicarboxylate | |
---|---|---|
Source | PubChem | |
URL | https://pubchem.ncbi.nlm.nih.gov | |
Description | Data deposited in or computed by PubChem | |
InChI |
InChI=1S/C10H12O5/c1-3-13-9(11)7-5-6-8(15-7)10(12)14-4-2/h5-6H,3-4H2,1-2H3 | |
Source | PubChem | |
URL | https://pubchem.ncbi.nlm.nih.gov | |
Description | Data deposited in or computed by PubChem | |
InChI Key |
PHGMGTWRSNXLDV-UHFFFAOYSA-N | |
Source | PubChem | |
URL | https://pubchem.ncbi.nlm.nih.gov | |
Description | Data deposited in or computed by PubChem | |
Canonical SMILES |
CCOC(=O)C1=CC=C(O1)C(=O)OCC | |
Source | PubChem | |
URL | https://pubchem.ncbi.nlm.nih.gov | |
Description | Data deposited in or computed by PubChem | |
Molecular Formula |
C10H12O5 | |
Source | PubChem | |
URL | https://pubchem.ncbi.nlm.nih.gov | |
Description | Data deposited in or computed by PubChem | |
DSSTOX Substance ID |
DTXSID90344506 | |
Record name | diethyl furan-2,5-dicarboxylate | |
Source | EPA DSSTox | |
URL | https://comptox.epa.gov/dashboard/DTXSID90344506 | |
Description | DSSTox provides a high quality public chemistry resource for supporting improved predictive toxicology. | |
Molecular Weight |
212.20 g/mol | |
Source | PubChem | |
URL | https://pubchem.ncbi.nlm.nih.gov | |
Description | Data deposited in or computed by PubChem | |
Product Name |
Diethyl furan-2,5-dicarboxylate | |
CAS RN |
53662-83-2 | |
Record name | diethyl furan-2,5-dicarboxylate | |
Source | EPA DSSTox | |
URL | https://comptox.epa.gov/dashboard/DTXSID90344506 | |
Description | DSSTox provides a high quality public chemistry resource for supporting improved predictive toxicology. | |
Record name | Diethyl furan-2,5-dicarboxylate | |
Source | European Chemicals Agency (ECHA) | |
URL | https://echa.europa.eu/information-on-chemicals | |
Description | The European Chemicals Agency (ECHA) is an agency of the European Union which is the driving force among regulatory authorities in implementing the EU's groundbreaking chemicals legislation for the benefit of human health and the environment as well as for innovation and competitiveness. | |
Explanation | Use of the information, documents and data from the ECHA website is subject to the terms and conditions of this Legal Notice, and subject to other binding limitations provided for under applicable law, the information, documents and data made available on the ECHA website may be reproduced, distributed and/or used, totally or in part, for non-commercial purposes provided that ECHA is acknowledged as the source: "Source: European Chemicals Agency, http://echa.europa.eu/". Such acknowledgement must be included in each copy of the material. ECHA permits and encourages organisations and individuals to create links to the ECHA website under the following cumulative conditions: Links can only be made to webpages that provide a link to the Legal Notice page. | |
Retrosynthesis Analysis
AI-Powered Synthesis Planning: Our tool employs the Template_relevance Pistachio, Template_relevance Bkms_metabolic, Template_relevance Pistachio_ringbreaker, Template_relevance Reaxys, Template_relevance Reaxys_biocatalysis model, leveraging a vast database of chemical reactions to predict feasible synthetic routes.
One-Step Synthesis Focus: Specifically designed for one-step synthesis, it provides concise and direct routes for your target compounds, streamlining the synthesis process.
Accurate Predictions: Utilizing the extensive PISTACHIO, BKMS_METABOLIC, PISTACHIO_RINGBREAKER, REAXYS, REAXYS_BIOCATALYSIS database, our tool offers high-accuracy predictions, reflecting the latest in chemical research and data.
Strategy Settings
Precursor scoring | Relevance Heuristic |
---|---|
Min. plausibility | 0.01 |
Model | Template_relevance |
Template Set | Pistachio/Bkms_metabolic/Pistachio_ringbreaker/Reaxys/Reaxys_biocatalysis |
Top-N result to add to graph | 6 |
Feasible Synthetic Routes
Q & A
Q1: What makes DEFDC an attractive alternative to petroleum-based monomers in polyester production?
A1: DEFDC is derived from renewable resources, specifically from the oxidation of 5-hydroxymethylfurfural (HMF), which can be obtained from biomass. [] This makes it a more sustainable alternative to petroleum-based monomers like terephthalic acid used in conventional polyesters. Furthermore, incorporating DEFDC into polyesters can lead to desirable material properties. For example, polyethylene furanoate (PEF), a polyester made from DEFDC and ethylene glycol, exhibits improved barrier properties compared to its petroleum-based counterpart, polyethylene terephthalate (PET). []
Q2: What are the challenges in using DEFDC for polyester synthesis, and how can they be addressed?
A2: One challenge lies in the reactivity of DEFDC with different diols, especially shorter ones like butane-1,4-diol. Research indicates that longer diols, starting from octane-1,8-diol, exhibit better reactivity with DEFDC, achieving up to 90% incorporation. [] To overcome the limitations with shorter diols, researchers have successfully utilized long-chain fatty dimer diols, such as Pripol 2033. [] This strategy allows for higher DEFDC incorporation and leads to polyesters with increased molecular weight.
Q3: What role does enzymatic catalysis play in DEFDC-based polyester synthesis?
A3: Enzymatic catalysis, specifically using immobilized lipase from Candida antarctica, offers a green and efficient approach for DEFDC polymerization with various aliphatic diols and diesters. [] This method allows for a controlled polymerization process and can be carried out in a two-step reaction using diphenyl ether as a solvent. [] The use of enzymes also aligns with the principles of green chemistry by reducing the reliance on harsh reaction conditions and toxic catalysts often employed in conventional polymerization methods.
Q4: How does varying the chain length of diols and diesters impact the properties of DEFDC-based polyesters?
A4: The choice of diol and diester significantly influences the final properties of the resulting polyester. For instance, shorter diols like hexane-1,6-diol show better reactivity towards longer diesters, while longer diols like dodecane-1,12-diol demonstrate reactivity with a wider range of diesters. [] Furthermore, incorporating DEFDC with diglycerol via a regioselective 1-OH acylation process allows for the synthesis of more soluble and hydrophilic furanic polyesters. [] This highlights the versatility of DEFDC in designing polymers with tailored properties for various applications.
Q5: What are the potential applications of DEFDC-based polyesters?
A5: The tunable properties of DEFDC-based polyesters make them promising candidates for diverse applications. Their improved barrier properties compared to conventional PET make them suitable for food packaging, potentially extending shelf life and reducing food waste. [] Additionally, their biobased origin and potential biodegradability position them as attractive alternatives in biomedical applications, where biocompatibility is crucial. Further research and development of DEFDC-based polyesters could unlock even more applications in areas ranging from textiles to coatings.
Disclaimer and Information on In-Vitro Research Products
Please be aware that all articles and product information presented on BenchChem are intended solely for informational purposes. The products available for purchase on BenchChem are specifically designed for in-vitro studies, which are conducted outside of living organisms. In-vitro studies, derived from the Latin term "in glass," involve experiments performed in controlled laboratory settings using cells or tissues. It is important to note that these products are not categorized as medicines or drugs, and they have not received approval from the FDA for the prevention, treatment, or cure of any medical condition, ailment, or disease. We must emphasize that any form of bodily introduction of these products into humans or animals is strictly prohibited by law. It is essential to adhere to these guidelines to ensure compliance with legal and ethical standards in research and experimentation.