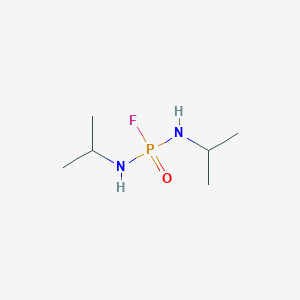
Mipafox
Overview
Description
Mipafox is a highly toxic organophosphate insecticide that acts as an irreversible acetylcholinesterase inhibitor. It was developed in the 1950s and is known for its resistance to cholinesterase reactivators . This compound is no longer in use due to its high toxicity and potential for causing delayed neurotoxicity and paralysis .
Preparation Methods
Mipafox is synthesized through a two-step process. First, phosphoryl chloride is reacted with isopropylamine to form an intermediate product. This intermediate is then reacted with potassium fluoride or ammonium fluoride to produce this compound . The reaction conditions typically involve controlled temperatures and the use of solvents to facilitate the reactions.
Chemical Reactions Analysis
Mipafox undergoes several types of chemical reactions, primarily involving its interaction with enzymes. The hydroxyl oxygen of the catalytic triad serine in the active center of serine hydrolase acetylcholinesterase attacks the phosphorus atom of this compound, displacing the primary leaving group and forming a covalent bond . This reaction is irreversible and leads to the inhibition of the enzyme. Common reagents used in these reactions include nucleophilic agents such as oximes, although this compound is resistant to reactivation by these agents . The major product formed from this reaction is a phosphorylated enzyme complex that is refractory to reactivation .
Scientific Research Applications
Mipafox has been extensively studied for its interactions with acetylcholinesterase and butyrylcholinesterase. These studies have provided insights into the mechanisms of enzyme inhibition and the aging process of phosphorylated enzymes . This compound has also been used as a model compound to study the effects of organophosphorus compounds on the nervous system and to develop antidotes for organophosphate poisoning . In addition, this compound has been used in theoretical studies to understand the phosphonylation and aging mechanisms of cholinesterases .
Mechanism of Action
Mipafox exerts its effects by inhibiting acetylcholinesterase, an enzyme responsible for hydrolyzing acetylcholine in the nervous system. The inhibition occurs through the formation of a covalent bond between the phosphorus atom of this compound and the hydroxyl oxygen of the catalytic triad serine in the active center of the enzyme . This bond is irreversible, leading to the accumulation of acetylcholine and subsequent overstimulation of cholinergic receptors . The aging process of the phosphorylated enzyme further stabilizes the inhibition, making it resistant to reactivation by nucleophilic agents .
Comparison with Similar Compounds
Mipafox is similar to other organophosphate compounds such as sarin and paraoxon, which also inhibit acetylcholinesterase through phosphorylation . this compound is unique in its resistance to reactivation by oximes and its potential for causing delayed neurotoxicity . Other similar compounds include dimefox and schradan, which share similar mechanisms of action but differ in their chemical structures and toxicological profiles .
Properties
IUPAC Name |
N-[fluoro-(propan-2-ylamino)phosphoryl]propan-2-amine | |
---|---|---|
Source | PubChem | |
URL | https://pubchem.ncbi.nlm.nih.gov | |
Description | Data deposited in or computed by PubChem | |
InChI |
InChI=1S/C6H16FN2OP/c1-5(2)8-11(7,10)9-6(3)4/h5-6H,1-4H3,(H2,8,9,10) | |
Source | PubChem | |
URL | https://pubchem.ncbi.nlm.nih.gov | |
Description | Data deposited in or computed by PubChem | |
InChI Key |
UOSHUBFBCPGQAY-UHFFFAOYSA-N | |
Source | PubChem | |
URL | https://pubchem.ncbi.nlm.nih.gov | |
Description | Data deposited in or computed by PubChem | |
Canonical SMILES |
CC(C)NP(=O)(NC(C)C)F | |
Source | PubChem | |
URL | https://pubchem.ncbi.nlm.nih.gov | |
Description | Data deposited in or computed by PubChem | |
Molecular Formula |
C6H16FN2OP | |
Source | PubChem | |
URL | https://pubchem.ncbi.nlm.nih.gov | |
Description | Data deposited in or computed by PubChem | |
DSSTOX Substance ID |
DTXSID5042160 | |
Record name | Mipafox | |
Source | EPA DSSTox | |
URL | https://comptox.epa.gov/dashboard/DTXSID5042160 | |
Description | DSSTox provides a high quality public chemistry resource for supporting improved predictive toxicology. | |
Molecular Weight |
182.18 g/mol | |
Source | PubChem | |
URL | https://pubchem.ncbi.nlm.nih.gov | |
Description | Data deposited in or computed by PubChem | |
Physical Description |
Odorless crystals; [HSDB] | |
Record name | Mipafox | |
Source | Haz-Map, Information on Hazardous Chemicals and Occupational Diseases | |
URL | https://haz-map.com/Agents/1140 | |
Description | Haz-Map® is an occupational health database designed for health and safety professionals and for consumers seeking information about the adverse effects of workplace exposures to chemical and biological agents. | |
Explanation | Copyright (c) 2022 Haz-Map(R). All rights reserved. Unless otherwise indicated, all materials from Haz-Map are copyrighted by Haz-Map(R). No part of these materials, either text or image may be used for any purpose other than for personal use. Therefore, reproduction, modification, storage in a retrieval system or retransmission, in any form or by any means, electronic, mechanical or otherwise, for reasons other than personal use, is strictly prohibited without prior written permission. | |
Boiling Point |
125 °C | |
Record name | MIPAFOX | |
Source | Hazardous Substances Data Bank (HSDB) | |
URL | https://pubchem.ncbi.nlm.nih.gov/source/hsdb/1585 | |
Description | The Hazardous Substances Data Bank (HSDB) is a toxicology database that focuses on the toxicology of potentially hazardous chemicals. It provides information on human exposure, industrial hygiene, emergency handling procedures, environmental fate, regulatory requirements, nanomaterials, and related areas. The information in HSDB has been assessed by a Scientific Review Panel. | |
Solubility |
Soluble in polar organic solvents and slightly soluble in petroleum ether., In water, 80,000 mg/L at 25 °C | |
Record name | MIPAFOX | |
Source | Hazardous Substances Data Bank (HSDB) | |
URL | https://pubchem.ncbi.nlm.nih.gov/source/hsdb/1585 | |
Description | The Hazardous Substances Data Bank (HSDB) is a toxicology database that focuses on the toxicology of potentially hazardous chemicals. It provides information on human exposure, industrial hygiene, emergency handling procedures, environmental fate, regulatory requirements, nanomaterials, and related areas. The information in HSDB has been assessed by a Scientific Review Panel. | |
Density |
1.2 at 25 °C | |
Record name | MIPAFOX | |
Source | Hazardous Substances Data Bank (HSDB) | |
URL | https://pubchem.ncbi.nlm.nih.gov/source/hsdb/1585 | |
Description | The Hazardous Substances Data Bank (HSDB) is a toxicology database that focuses on the toxicology of potentially hazardous chemicals. It provides information on human exposure, industrial hygiene, emergency handling procedures, environmental fate, regulatory requirements, nanomaterials, and related areas. The information in HSDB has been assessed by a Scientific Review Panel. | |
Vapor Pressure |
0.001 [mmHg], 0.001 mm Hg at 15 °C | |
Record name | Mipafox | |
Source | Haz-Map, Information on Hazardous Chemicals and Occupational Diseases | |
URL | https://haz-map.com/Agents/1140 | |
Description | Haz-Map® is an occupational health database designed for health and safety professionals and for consumers seeking information about the adverse effects of workplace exposures to chemical and biological agents. | |
Explanation | Copyright (c) 2022 Haz-Map(R). All rights reserved. Unless otherwise indicated, all materials from Haz-Map are copyrighted by Haz-Map(R). No part of these materials, either text or image may be used for any purpose other than for personal use. Therefore, reproduction, modification, storage in a retrieval system or retransmission, in any form or by any means, electronic, mechanical or otherwise, for reasons other than personal use, is strictly prohibited without prior written permission. | |
Record name | MIPAFOX | |
Source | Hazardous Substances Data Bank (HSDB) | |
URL | https://pubchem.ncbi.nlm.nih.gov/source/hsdb/1585 | |
Description | The Hazardous Substances Data Bank (HSDB) is a toxicology database that focuses on the toxicology of potentially hazardous chemicals. It provides information on human exposure, industrial hygiene, emergency handling procedures, environmental fate, regulatory requirements, nanomaterials, and related areas. The information in HSDB has been assessed by a Scientific Review Panel. | |
Mechanism of Action |
...Cholinesterase inhibitor., The neurotoxic esterase fraction of hen brain microsomal phenyl-valerate-hydrolyzing activity is the target for mipafox., A direct method of assaying neurotoxic esterase (NTE) activity, using 4-nitrophenyl valerate, was described. The technique was used to determine the bimolecular rate, phosphorylation and affinity constants for the reaction of chicken brain microsomal NTE with mipafox., A number of... compounds such as... Mipafox... have the ability to bind tenaciously to the active site of acetylcholinesterase (AChE) and neuropathy target esterase (NTE) to produce an irreversibly inhibited enzyme by a mechanism known as aging. The aging process is dependent on the size and configuration of the alkyl (R) substituent, with the potency of the ester increasing in the order of diethyl, dipropyl, and dibutyl for such analogs as DFP and mipafox. The aging process is generally accepted as being caused by the dealkylation of the intermediate dialkylphosphorylated enzymes by one of two possible mechanisms. The first involves the hydrolysis of a P-O bond following a nucleophile (base) attack on the phosphorus atom. The second mechanism involves the hydrolysis of an O-C bond by an acid catalysis, resulting in the formation of a carbonium ion as the leaving group. The aging process is believed to fix an extra charge to the protein, causing some perturbation to the active site and thereby preventing dephosphorylation. While the exact nature of this reaction has not been demonstrated for AChE and NTE, evidence from experiments with saligenin cyclic phosphorus esters (derivative of TOTP) and alpha-chymotrypsin points to the possibility of two stabilized forms of "aged" enzyme. Both of the reactions utilize the imidazole group of a neighboring histidine. In one reaction, the hydroxylated substituent is released and the phosphorylated enzyme is stabilized by a hydrogen on the imidazole group. In the other reaction, the leaving substituent becomes attached to the imidazole, yielding a N-C hydroxylated derivative of the phosphorylated enzyme. | |
Record name | MIPAFOX | |
Source | Hazardous Substances Data Bank (HSDB) | |
URL | https://pubchem.ncbi.nlm.nih.gov/source/hsdb/1585 | |
Description | The Hazardous Substances Data Bank (HSDB) is a toxicology database that focuses on the toxicology of potentially hazardous chemicals. It provides information on human exposure, industrial hygiene, emergency handling procedures, environmental fate, regulatory requirements, nanomaterials, and related areas. The information in HSDB has been assessed by a Scientific Review Panel. | |
Impurities |
Main impurities of commercial product are hydrolytic products & the Isopropylamide of orthophosphoric acid... | |
Record name | MIPAFOX | |
Source | Hazardous Substances Data Bank (HSDB) | |
URL | https://pubchem.ncbi.nlm.nih.gov/source/hsdb/1585 | |
Description | The Hazardous Substances Data Bank (HSDB) is a toxicology database that focuses on the toxicology of potentially hazardous chemicals. It provides information on human exposure, industrial hygiene, emergency handling procedures, environmental fate, regulatory requirements, nanomaterials, and related areas. The information in HSDB has been assessed by a Scientific Review Panel. | |
Color/Form |
Crystals from petroleum ether | |
CAS No. |
371-86-8 | |
Record name | Mipafox | |
Source | CAS Common Chemistry | |
URL | https://commonchemistry.cas.org/detail?cas_rn=371-86-8 | |
Description | CAS Common Chemistry is an open community resource for accessing chemical information. Nearly 500,000 chemical substances from CAS REGISTRY cover areas of community interest, including common and frequently regulated chemicals, and those relevant to high school and undergraduate chemistry classes. This chemical information, curated by our expert scientists, is provided in alignment with our mission as a division of the American Chemical Society. | |
Explanation | The data from CAS Common Chemistry is provided under a CC-BY-NC 4.0 license, unless otherwise stated. | |
Record name | Mipafox [ISO] | |
Source | ChemIDplus | |
URL | https://pubchem.ncbi.nlm.nih.gov/substance/?source=chemidplus&sourceid=0000371868 | |
Description | ChemIDplus is a free, web search system that provides access to the structure and nomenclature authority files used for the identification of chemical substances cited in National Library of Medicine (NLM) databases, including the TOXNET system. | |
Record name | MIPAFOX | |
Source | DTP/NCI | |
URL | https://dtp.cancer.gov/dtpstandard/servlet/dwindex?searchtype=NSC&outputformat=html&searchlist=8924 | |
Description | The NCI Development Therapeutics Program (DTP) provides services and resources to the academic and private-sector research communities worldwide to facilitate the discovery and development of new cancer therapeutic agents. | |
Explanation | Unless otherwise indicated, all text within NCI products is free of copyright and may be reused without our permission. Credit the National Cancer Institute as the source. | |
Record name | Mipafox | |
Source | EPA DSSTox | |
URL | https://comptox.epa.gov/dashboard/DTXSID5042160 | |
Description | DSSTox provides a high quality public chemistry resource for supporting improved predictive toxicology. | |
Record name | Mipafox | |
Source | European Chemicals Agency (ECHA) | |
URL | https://echa.europa.eu/substance-information/-/substanceinfo/100.006.130 | |
Description | The European Chemicals Agency (ECHA) is an agency of the European Union which is the driving force among regulatory authorities in implementing the EU's groundbreaking chemicals legislation for the benefit of human health and the environment as well as for innovation and competitiveness. | |
Explanation | Use of the information, documents and data from the ECHA website is subject to the terms and conditions of this Legal Notice, and subject to other binding limitations provided for under applicable law, the information, documents and data made available on the ECHA website may be reproduced, distributed and/or used, totally or in part, for non-commercial purposes provided that ECHA is acknowledged as the source: "Source: European Chemicals Agency, http://echa.europa.eu/". Such acknowledgement must be included in each copy of the material. ECHA permits and encourages organisations and individuals to create links to the ECHA website under the following cumulative conditions: Links can only be made to webpages that provide a link to the Legal Notice page. | |
Record name | MIPAFOX | |
Source | FDA Global Substance Registration System (GSRS) | |
URL | https://gsrs.ncats.nih.gov/ginas/app/beta/substances/24MJP5H3YN | |
Description | The FDA Global Substance Registration System (GSRS) enables the efficient and accurate exchange of information on what substances are in regulated products. Instead of relying on names, which vary across regulatory domains, countries, and regions, the GSRS knowledge base makes it possible for substances to be defined by standardized, scientific descriptions. | |
Explanation | Unless otherwise noted, the contents of the FDA website (www.fda.gov), both text and graphics, are not copyrighted. They are in the public domain and may be republished, reprinted and otherwise used freely by anyone without the need to obtain permission from FDA. Credit to the U.S. Food and Drug Administration as the source is appreciated but not required. | |
Record name | MIPAFOX | |
Source | Hazardous Substances Data Bank (HSDB) | |
URL | https://pubchem.ncbi.nlm.nih.gov/source/hsdb/1585 | |
Description | The Hazardous Substances Data Bank (HSDB) is a toxicology database that focuses on the toxicology of potentially hazardous chemicals. It provides information on human exposure, industrial hygiene, emergency handling procedures, environmental fate, regulatory requirements, nanomaterials, and related areas. The information in HSDB has been assessed by a Scientific Review Panel. | |
Melting Point |
65 °C | |
Record name | MIPAFOX | |
Source | Hazardous Substances Data Bank (HSDB) | |
URL | https://pubchem.ncbi.nlm.nih.gov/source/hsdb/1585 | |
Description | The Hazardous Substances Data Bank (HSDB) is a toxicology database that focuses on the toxicology of potentially hazardous chemicals. It provides information on human exposure, industrial hygiene, emergency handling procedures, environmental fate, regulatory requirements, nanomaterials, and related areas. The information in HSDB has been assessed by a Scientific Review Panel. | |
Retrosynthesis Analysis
AI-Powered Synthesis Planning: Our tool employs the Template_relevance Pistachio, Template_relevance Bkms_metabolic, Template_relevance Pistachio_ringbreaker, Template_relevance Reaxys, Template_relevance Reaxys_biocatalysis model, leveraging a vast database of chemical reactions to predict feasible synthetic routes.
One-Step Synthesis Focus: Specifically designed for one-step synthesis, it provides concise and direct routes for your target compounds, streamlining the synthesis process.
Accurate Predictions: Utilizing the extensive PISTACHIO, BKMS_METABOLIC, PISTACHIO_RINGBREAKER, REAXYS, REAXYS_BIOCATALYSIS database, our tool offers high-accuracy predictions, reflecting the latest in chemical research and data.
Strategy Settings
Precursor scoring | Relevance Heuristic |
---|---|
Min. plausibility | 0.01 |
Model | Template_relevance |
Template Set | Pistachio/Bkms_metabolic/Pistachio_ringbreaker/Reaxys/Reaxys_biocatalysis |
Top-N result to add to graph | 6 |
Feasible Synthetic Routes
Disclaimer and Information on In-Vitro Research Products
Please be aware that all articles and product information presented on BenchChem are intended solely for informational purposes. The products available for purchase on BenchChem are specifically designed for in-vitro studies, which are conducted outside of living organisms. In-vitro studies, derived from the Latin term "in glass," involve experiments performed in controlled laboratory settings using cells or tissues. It is important to note that these products are not categorized as medicines or drugs, and they have not received approval from the FDA for the prevention, treatment, or cure of any medical condition, ailment, or disease. We must emphasize that any form of bodily introduction of these products into humans or animals is strictly prohibited by law. It is essential to adhere to these guidelines to ensure compliance with legal and ethical standards in research and experimentation.