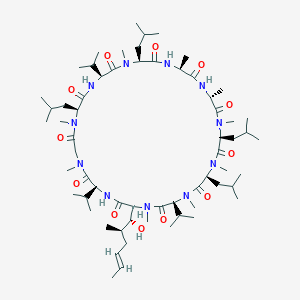
Cyclosporin D
Overview
Description
Cyclosporin D is a cyclic undecapeptide, a member of the cyclosporin family, known for its immunosuppressive properties It is derived from the fungus Tolypocladium inflatum and is structurally similar to other cyclosporins, such as cyclosporin A
Mechanism of Action
Target of Action
Cyclosporin D primarily targets T cells . It interacts with the receptor cyclophilin-1 inside cells . This interaction is crucial for the compound’s immunosuppressive action .
Mode of Action
This compound forms a complex known as cyclosporine-cyclophilin upon binding to cyclophilin-1 . This complex subsequently inhibits calcineurin , which in turn stops the dephosphorylation as well as the activation of the nuclear factor of activated T-cells (NF-AT), a transcription factor . This inhibition blocks T cell-dependent biosynthesis of lymphokines, particularly interleukin 2 , at the level of messenger ribonucleic acid (mRNA) transcription .
Biochemical Pathways
This compound affects the calcineurin-NF-AT pathway . By inhibiting calcineurin, it prevents the activation of NF-AT, which is crucial for the transcription of interleukin 2 . This results in the selective inhibition of adaptive immune responses . Recent studies also show that this compound blocks both p38 and c-Jun N-terminal kinase (JNK) pathways in addition to calcineurin blocking activity .
Pharmacokinetics
This compound exhibits low oral bioavailability due to its high molecular weight and low solubility . It is metabolized in the liver by P-450 enzymes , and drugs that affect the P-450 system also affect the metabolism of this compound . It is extensively distributed to extravascular tissues because of its high lipid solubility .
Result of Action
The primary result of this compound’s action is immunosuppression . By inhibiting the production of interleukin 2, it suppresses T cell activation and proliferation . This makes it effective in preventing organ transplant rejection and treating various inflammatory and autoimmune conditions .
Action Environment
The action of this compound can be influenced by various environmental factors. For instance, its absorption and metabolism can be affected by the presence of other drugs that interact with the P-450 enzyme system . Additionally, minor variations in the structure of its amino acid components can reduce its immunosuppressive activity .
Biochemical Analysis
Biochemical Properties
Cyclosporin D interacts with various enzymes, proteins, and other biomolecules. It is well known for being the target of the immunosuppressive drug cyclosporin, used to combat organ transplant rejection . The cyclophilin family of peptidyl-prolyl isomerases, which catalyze the conversion between cis and trans isomers of proline, is a notable target of this compound .
Cellular Effects
This compound has significant effects on various types of cells and cellular processes. It reduces the production of a range of cytokines, inhibiting the activation and/or maturation of various cell types, including those involved in cell-mediated immunity . This explains its role in the prevention of graft rejection.
Molecular Mechanism
This compound exerts its effects at the molecular level through several mechanisms. It produces calcium-dependent, specific, reversible inhibition of transcription of interleukin-2 and several other cytokines, most notably in T helper lymphocytes . This inhibition is key to its immunosuppressive properties.
Temporal Effects in Laboratory Settings
The effects of this compound change over time in laboratory settings. For example, changes in the dose given and the creatinine concentration were highly significant . Rejection rates were similar, but a comparison of clinical benefit showed the best results when samples for cyclosporin measurement were taken at 2 h post-dose .
Dosage Effects in Animal Models
The effects of this compound vary with different dosages in animal models. Low doses appear to have immunomodulatory properties, with different effects from high doses on CD8+ T lymphocyte activation, auto-immune diseases, graft-vs.-host disease, and cancer .
Metabolic Pathways
This compound is involved in several metabolic pathways. It is metabolized in the intestine and the liver by CYP450 enzymes, predominantly CYP3A4 with contributions from CYP3A5 .
Transport and Distribution
This compound is transported and distributed within cells and tissues. A considerable proportion of cyclosporin synthetase and D-alanine racemase was detected at the vacuolar membrane . The product cyclosporin was localized in the fungal vacuole .
Subcellular Localization
The subcellular localization of this compound and its effects on its activity or function are significant. The electron microscopic image of native cyclosporin synthetase molecules showed large globular complexes of 25 nm in diameter, built up by smaller interconnected units . A considerable proportion of cyclosporin synthetase and D-alanine racemase was detected at the vacuolar membrane .
Preparation Methods
Synthetic Routes and Reaction Conditions: Cyclosporin D can be synthesized chemically using N-methylated peptides via a series of isonitrile coupling reactions. The synthesis involves the preparation of the enantiomerically pure amino acid (4R)-4-((E)-2-butenyl-4,N-dimethyl-L-threonine), which is a key component of the cyclosporin structure .
Industrial Production Methods: Industrial production of this compound typically involves fermentation processes using the fungus Tolypocladium inflatum. The fermentation broth is then subjected to extraction and purification processes to isolate the desired compound .
Chemical Reactions Analysis
Types of Reactions: Cyclosporin D undergoes various chemical reactions, including:
Oxidation: this compound can be oxidized to form different metabolites.
Reduction: Reduction reactions can modify the structure of this compound, affecting its biological activity.
Substitution: Substitution reactions can introduce different functional groups into the this compound molecule, potentially altering its properties.
Common Reagents and Conditions:
Oxidation: Common oxidizing agents include hydrogen peroxide and potassium permanganate.
Reduction: Reducing agents such as sodium borohydride and lithium aluminum hydride are used.
Substitution: Various reagents, including halogens and alkylating agents, are employed in substitution reactions.
Major Products Formed: The major products formed from these reactions depend on the specific conditions and reagents used. For example, oxidation can lead to the formation of hydroxylated metabolites, while reduction can produce dehydrogenated derivatives .
Scientific Research Applications
Cyclosporin D has a wide range of scientific research applications, including:
Chemistry: Used as a model compound for studying peptide synthesis and structure-activity relationships.
Biology: Investigated for its effects on cellular processes and signaling pathways.
Medicine: Explored for its potential in preventing organ transplant rejection and treating autoimmune diseases.
Industry: Utilized in the development of novel drug delivery systems and formulations.
Comparison with Similar Compounds
Cyclosporin A: The most well-known member of the cyclosporin family, widely used in clinical practice.
Cyclosporin B: Another member of the cyclosporin family with similar immunosuppressive properties.
Cyclosporin C:
Uniqueness of Cyclosporin D: this compound is unique due to its specific amino acid composition and structural features, which contribute to its distinct biological activity. Its selective inhibition of calcineurin and interaction with cyclophilin make it a valuable compound for research and therapeutic applications .
Properties
IUPAC Name |
33-(1-hydroxy-2-methylhex-4-enyl)-1,4,7,10,12,15,19,25,28-nonamethyl-6,9,18,24-tetrakis(2-methylpropyl)-3,21,30-tri(propan-2-yl)-1,4,7,10,13,16,19,22,25,28,31-undecazacyclotritriacontane-2,5,8,11,14,17,20,23,26,29,32-undecone | |
---|---|---|
Details | Computed by LexiChem 2.6.6 (PubChem release 2019.06.18) | |
Source | PubChem | |
URL | https://pubchem.ncbi.nlm.nih.gov | |
Description | Data deposited in or computed by PubChem | |
InChI |
InChI=1S/C63H113N11O12/c1-26-27-28-41(16)53(76)52-57(80)67-49(38(10)11)61(84)68(19)33-48(75)69(20)44(29-34(2)3)56(79)66-50(39(12)13)62(85)70(21)45(30-35(4)5)55(78)64-42(17)54(77)65-43(18)58(81)71(22)46(31-36(6)7)59(82)72(23)47(32-37(8)9)60(83)73(24)51(40(14)15)63(86)74(52)25/h26-27,34-47,49-53,76H,28-33H2,1-25H3,(H,64,78)(H,65,77)(H,66,79)(H,67,80) | |
Details | Computed by InChI 1.0.5 (PubChem release 2019.06.18) | |
Source | PubChem | |
URL | https://pubchem.ncbi.nlm.nih.gov | |
Description | Data deposited in or computed by PubChem | |
InChI Key |
ZNVBEWJRWHNZMK-UHFFFAOYSA-N | |
Details | Computed by InChI 1.0.5 (PubChem release 2019.06.18) | |
Source | PubChem | |
URL | https://pubchem.ncbi.nlm.nih.gov | |
Description | Data deposited in or computed by PubChem | |
Canonical SMILES |
CC=CCC(C)C(C1C(=O)NC(C(=O)N(CC(=O)N(C(C(=O)NC(C(=O)N(C(C(=O)NC(C(=O)NC(C(=O)N(C(C(=O)N(C(C(=O)N(C(C(=O)N1C)C(C)C)C)CC(C)C)C)CC(C)C)C)C)C)CC(C)C)C)C(C)C)CC(C)C)C)C)C(C)C)O | |
Details | Computed by OEChem 2.1.5 (PubChem release 2019.06.18) | |
Source | PubChem | |
URL | https://pubchem.ncbi.nlm.nih.gov | |
Description | Data deposited in or computed by PubChem | |
Molecular Formula |
C63H113N11O12 | |
Details | Computed by PubChem 2.1 (PubChem release 2019.06.18) | |
Source | PubChem | |
URL | https://pubchem.ncbi.nlm.nih.gov | |
Description | Data deposited in or computed by PubChem | |
Molecular Weight |
1216.6 g/mol | |
Details | Computed by PubChem 2.1 (PubChem release 2021.05.07) | |
Source | PubChem | |
URL | https://pubchem.ncbi.nlm.nih.gov | |
Description | Data deposited in or computed by PubChem | |
CAS No. |
63775-96-2 | |
Record name | cyclosporin d | |
Source | European Chemicals Agency (ECHA) | |
URL | https://echa.europa.eu/information-on-chemicals | |
Description | The European Chemicals Agency (ECHA) is an agency of the European Union which is the driving force among regulatory authorities in implementing the EU's groundbreaking chemicals legislation for the benefit of human health and the environment as well as for innovation and competitiveness. | |
Explanation | Use of the information, documents and data from the ECHA website is subject to the terms and conditions of this Legal Notice, and subject to other binding limitations provided for under applicable law, the information, documents and data made available on the ECHA website may be reproduced, distributed and/or used, totally or in part, for non-commercial purposes provided that ECHA is acknowledged as the source: "Source: European Chemicals Agency, http://echa.europa.eu/". Such acknowledgement must be included in each copy of the material. ECHA permits and encourages organisations and individuals to create links to the ECHA website under the following cumulative conditions: Links can only be made to webpages that provide a link to the Legal Notice page. | |
Retrosynthesis Analysis
AI-Powered Synthesis Planning: Our tool employs the Template_relevance Pistachio, Template_relevance Bkms_metabolic, Template_relevance Pistachio_ringbreaker, Template_relevance Reaxys, Template_relevance Reaxys_biocatalysis model, leveraging a vast database of chemical reactions to predict feasible synthetic routes.
One-Step Synthesis Focus: Specifically designed for one-step synthesis, it provides concise and direct routes for your target compounds, streamlining the synthesis process.
Accurate Predictions: Utilizing the extensive PISTACHIO, BKMS_METABOLIC, PISTACHIO_RINGBREAKER, REAXYS, REAXYS_BIOCATALYSIS database, our tool offers high-accuracy predictions, reflecting the latest in chemical research and data.
Strategy Settings
Precursor scoring | Relevance Heuristic |
---|---|
Min. plausibility | 0.01 |
Model | Template_relevance |
Template Set | Pistachio/Bkms_metabolic/Pistachio_ringbreaker/Reaxys/Reaxys_biocatalysis |
Top-N result to add to graph | 6 |
Feasible Synthetic Routes
A: CsD binds to Pgp, inhibiting its drug efflux function and restoring the intracellular accumulation of chemotherapeutic agents. [, , , ] This interaction prevents Pgp from pumping drugs out of the cell, allowing the chemotherapeutics to reach cytotoxic concentrations and exert their intended effects. [, , ]
A: Studies show that CsD does not directly reduce the expression levels of Pgp mRNA or protein. [, ] Its primary mechanism of action involves functional inhibition of the transporter, rather than downregulating its production. [, ]
A: Both CsA and CsD are cyclic oligopeptides composed of 11 amino acids. The key structural difference lies in the amino acid at position 2: CsA contains L-α-aminobutyric acid, while CsD has L-valine in this position. []
A: While the provided research does not delve into detailed spectroscopic data for CsD, structural characterization was initially achieved through chemical investigation, spectroscopic evidence, and X-ray analysis. []
A: Research indicates that subtle changes in the cyclosporin structure can significantly influence their ability to inhibit Pgp. For instance, the 3’-keto derivative of CsD, valspodar (PSC 833), exhibits even greater potency in reversing multidrug resistance compared to CsD. [, , ] This suggests that specific structural motifs are crucial for optimal interaction with Pgp. [, ]
A: Yes, CsD has shown promising results in both in vitro and in vivo studies. It effectively reverses daunorubicin resistance in human leukemic cell lines by increasing intracellular drug accumulation and decreasing efflux. [] Additionally, CsD potentiates the antitumor activity of adriamycin, vincristine, and etoposide in mice bearing leukemia and solid tumors. []
A: Research highlights the emergence of a CsD-resistant human sarcoma cell line (DxP) during co-selection with doxorubicin and CsD. [] These cells exhibited a mutated Pgp with decreased affinity for CsD and altered drug resistance profiles. [] This underscores the potential for acquired resistance and emphasizes the need for strategies to mitigate this challenge. []
A: High-performance liquid chromatography (HPLC) coupled with various detection methods, including ultraviolet (UV) detection and mass spectrometry (MS), is widely used for CsD quantification in biological samples. [, , , , , , , , , , , ] Internal standards, like CsA, are frequently employed to ensure accuracy and precision. [, , , , , , , , , , , , ]
A: Yes, several compounds besides CsD can inhibit Pgp, including valspodar (PSC 833), verapamil, and quinidine. [, , , , , ] These agents exhibit varying potencies and may have distinct advantages and disadvantages in specific clinical settings. [, , , , ] The choice of the most appropriate Pgp inhibitor depends on factors such as the specific cancer type, drug resistance profile, and patient-specific factors.
Disclaimer and Information on In-Vitro Research Products
Please be aware that all articles and product information presented on BenchChem are intended solely for informational purposes. The products available for purchase on BenchChem are specifically designed for in-vitro studies, which are conducted outside of living organisms. In-vitro studies, derived from the Latin term "in glass," involve experiments performed in controlled laboratory settings using cells or tissues. It is important to note that these products are not categorized as medicines or drugs, and they have not received approval from the FDA for the prevention, treatment, or cure of any medical condition, ailment, or disease. We must emphasize that any form of bodily introduction of these products into humans or animals is strictly prohibited by law. It is essential to adhere to these guidelines to ensure compliance with legal and ethical standards in research and experimentation.