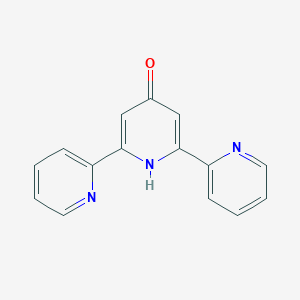
2,6-Bis(2-pyridyl)-4(1H)-pyridone
Overview
Description
2,6-Bis(2-pyridyl)-4(1H)-pyridone is a heterocyclic compound that features a pyridone core substituted with two pyridyl groups at the 2 and 6 positions
Preparation Methods
Synthetic Routes and Reaction Conditions
The synthesis of 2,6-Bis(2-pyridyl)-4(1H)-pyridone typically involves the condensation of 2-acetylpyridine with 2-pyridinecarboxaldehyde in the presence of a base, followed by cyclization. The reaction conditions often include the use of solvents such as ethanol or methanol and bases like sodium hydroxide or potassium carbonate.
Industrial Production Methods
While specific industrial production methods for this compound are not well-documented, the general approach would involve scaling up the laboratory synthesis methods. This would include optimizing reaction conditions to maximize yield and purity, as well as implementing continuous flow processes to enhance efficiency.
Chemical Reactions Analysis
Types of Reactions
2,6-Bis(2-pyridyl)-4(1H)-pyridone can undergo various chemical reactions, including:
Oxidation: The pyridone ring can be oxidized under strong oxidative conditions.
Reduction: The compound can be reduced to form dihydropyridone derivatives.
Substitution: The pyridyl groups can participate in electrophilic and nucleophilic substitution reactions.
Common Reagents and Conditions
Oxidation: Reagents such as potassium permanganate or chromium trioxide in acidic conditions.
Reduction: Reagents like sodium borohydride or lithium aluminum hydride.
Substitution: Conditions vary depending on the substituent being introduced, but common reagents include halogens, alkylating agents, and nucleophiles.
Major Products
Oxidation: Oxidized derivatives of the pyridone ring.
Reduction: Dihydropyridone derivatives.
Substitution: Various substituted pyridyl-pyridone compounds.
Scientific Research Applications
Coordination Chemistry
BPP is primarily known for its role as a ligand in coordination chemistry. It forms stable complexes with various transition metals, which are crucial for catalysis and materials science.
Applications in Catalysis
- BPP complexes have been studied for their catalytic activity in various organic transformations, including oxidation and reduction reactions. For instance, cobalt(II) complexes of BPP have shown promising results in spin crossover phenomena, which can be exploited in developing advanced materials for sensors and switches .
Metal-Organic Frameworks (MOFs)
- The ability of BPP to coordinate with metal ions makes it an excellent candidate for constructing MOFs. These frameworks have diverse topologies and functionalities, making them suitable for gas adsorption and separation applications.
Biological Studies
BPP's chelating properties enable it to interact effectively with metal ions, which is significant in biological systems.
Metalloprotein Studies
- Research has indicated that BPP can serve as a model compound for studying metalloproteins and metalloenzymes. Its interactions with metal ions are crucial for understanding enzyme mechanisms and developing inhibitors .
Potential Therapeutic Applications
- Ongoing investigations are exploring BPP's potential as a therapeutic agent in metal ion chelation therapy, particularly for conditions related to metal toxicity or deficiency. Preliminary studies suggest its efficacy against various cancer cell lines, indicating its potential as an antitumor agent.
Material Science
BPP's unique properties make it valuable in the development of new materials.
Luminescent Materials
- The compound exhibits photoluminescent properties, making it a candidate for organic light-emitting diodes (OLEDs) and sensors. Its ability to absorb light and emit at different wavelengths is being explored for various applications in optoelectronics .
Development of Functional Materials
- Researchers are investigating BPP's role in synthesizing functional materials with specific electronic or optical properties. Its dual functionality allows for the design of materials with tailored characteristics for advanced applications.
Summary of Applications
Application Area | Description | Examples/Case Studies |
---|---|---|
Coordination Chemistry | Ligand formation with transition metals for catalysis | Cobalt(II) spin crossover systems |
Biological Studies | Chelation of metal ions; potential antitumor activity | Interaction studies with metalloproteins |
Material Science | Development of MOFs; luminescent materials | OLEDs and gas adsorption systems |
Therapeutic Applications | Metal ion chelation therapy; cancer treatment potential | Studies on cancer cell lines |
Mechanism of Action
The mechanism by which 2,6-Bis(2-pyridyl)-4(1H)-pyridone exerts its effects is primarily through its ability to coordinate with metal ions. The pyridyl and pyridone groups can donate electron pairs to metal centers, forming stable complexes. These complexes can then participate in various catalytic and electronic processes, depending on the nature of the metal and the surrounding ligands.
Comparison with Similar Compounds
Similar Compounds
2,2’-Bipyridine: Another ligand with two pyridyl groups, but without the pyridone core.
1,10-Phenanthroline: A ligand with a similar coordination ability but a different ring structure.
2,6-Di(2-pyridyl)pyridine: Similar structure but lacks the pyridone functionality.
Uniqueness
2,6-Bis(2-pyridyl)-4(1H)-pyridone is unique due to the presence of both pyridyl and pyridone groups, which provide a versatile coordination environment. This allows for the formation of a wide range of metal complexes with varying properties, making it a valuable compound in coordination chemistry and related fields.
Biological Activity
2,6-Bis(2-pyridyl)-4(1H)-pyridone is a compound of significant interest in medicinal chemistry due to its diverse biological activities. This article explores its biological activity, focusing on cytotoxicity, antimalarial properties, and potential as a therapeutic agent against various diseases.
Chemical Structure and Properties
The chemical structure of this compound features two pyridyl groups attached to a central 4(1H)-pyridone moiety. This arrangement contributes to its ability to interact with biological macromolecules, particularly DNA.
Cytotoxicity Studies
Cytotoxicity assays have demonstrated that this compound exhibits significant cytotoxic effects against various cancer cell lines.
Table 1: Cytotoxicity of this compound on Different Cell Lines
Cell Line | IC50 (µM) | Reference |
---|---|---|
HCT116 (Colorectal) | 15.0 | |
GM05757 (Fibroblast) | 40.0 | |
A375 (Melanoma) | 20.5 | |
SK-Hep1 (Liver Cancer) | 12.3 |
The compound displayed an IC50 value of 15 µM against HCT116 cells, indicating potent cytotoxic activity. In comparison, it showed less toxicity towards normal fibroblast cells (IC50 = 40 µM), suggesting a degree of selectivity for cancerous cells.
The mechanism by which this compound exerts its cytotoxic effects involves the intercalation into DNA and the generation of reactive oxygen species (ROS). This leads to DNA strand breaks and subsequent activation of apoptotic pathways.
Case Study: Mechanistic Insights
In a study focused on the compound's interaction with DNA, it was observed that treatment with this compound resulted in increased phosphorylation of p53 and upregulation of p21, leading to G2/M cell cycle arrest and apoptosis in A375 melanoma cells .
Antimalarial Activity
Recent research has highlighted the potential of this compound as an antimalarial agent. The compound demonstrated activity against Plasmodium species in vitro.
Table 2: Antimalarial Activity of this compound
The compound exhibited an ED50 value of 0.6 mg/kg in murine models infected with Plasmodium yoelii, indicating strong efficacy as an antimalarial agent.
Additional Biological Activities
Beyond its cytotoxic and antimalarial properties, studies have suggested that this compound may possess antiviral properties and could be explored for use in treating viral infections .
Q & A
Basic Research Questions
Q. How is 2,6-Bis(2-pyridyl)-4(1H)-pyridone synthesized, and what analytical methods are used for characterization?
The ligand is synthesized via a double Claisen condensation of methyl quinaldate with acetone in the presence of sodium t-butoxide, followed by cyclization with ammonium acetate in methanol . Characterization involves NMR spectroscopy to resolve tautomeric forms (pyridine-pyridone equilibrium) and X-ray crystallography refined using programs like SHELX for structural elucidation . Purity is assessed via HPLC and mass spectrometry .
Q. What are the common coordination modes of this ligand with transition metals?
The ligand exhibits versatile coordination:
- Tridentate N^N^N binding (e.g., in Ag(I) complexes forming double-helical Ag₂N₆ cores) .
- Bidentate N^N or bridging modes (e.g., in Co(II) spin-crossover complexes) .
- Mixed N^N:O bonding in supramolecular assemblies .
Q. What are its primary applications in materials science?
- Building block for 4′-substituted terpyridines via Mitsunobu reactions with alcohols or nucleosides .
- Preparation of cyclotriphosphazene ligands with pendant terpyridine moieties for catalysis or photophysics .
- Component in metal-organic frameworks (MOFs) and covalent organic frameworks (COFs) for functional materials .
Advanced Research Questions
Q. How does tautomerism affect spectroscopic and structural analyses?
The ligand exists in equilibrium between pyridine and pyridone tautomers , complicating NMR interpretation. Theoretical calculations (e.g., DFT) and variable-temperature NMR are used to resolve tautomeric populations. X-ray crystallography with SHELX refinement confirms dominant tautomeric forms in the solid state .
Q. What mechanistic insights explain fluorescence shifts upon metal coordination?
Coordination with Ag(I) induces a blue shift due to stabilization of mixed MLCT/ILCT (metal-to-ligand/ligand-centered charge transfer) excited states. Emission originates from a singlet state , confirmed by lifetime measurements and computational modeling .
Q. How can experimental contradictions in magnetic properties be resolved?
Discrepancies in spin-crossover behavior (e.g., Co(II) complexes) arise from solvent effects or ligand-field variations. Controlled desorption of crystal solvents and magnetic susceptibility studies under inert conditions clarify these anomalies .
Q. What strategies optimize the synthesis of derivatives via Mitsunobu reactions?
Replacing hazardous sodium hydride with sodium t-butoxide improves safety and yield (>90%) in Mitsunobu reactions. Steric and electronic effects of ω-substituted alcohols are critical for regioselectivity .
Q. What challenges arise in crystallizing this ligand, and how are they addressed?
Low solubility in common solvents necessitates mixed solvent systems (e.g., THF/MeOH). Microcrystalline powders often require high-resolution data and SHELX refinement for accurate structure determination .
Q. How does ligand substitution (e.g., quinoline vs. pyridine) alter photophysical properties?
Substituting pyridine with quinoline extends conjugation, red-shifting absorption/emission by ~50 nm. This is leveraged in designing near-IR emitters for sensing applications .
Q. What methodologies validate ligand purity in supramolecular assemblies?
High-resolution mass spectrometry (HRMS) and elemental analysis confirm stoichiometry. Purity thresholds (>98%) are essential to avoid competing coordination modes in self-assembly .
Properties
IUPAC Name |
2,6-dipyridin-2-yl-1H-pyridin-4-one | |
---|---|---|
Source | PubChem | |
URL | https://pubchem.ncbi.nlm.nih.gov | |
Description | Data deposited in or computed by PubChem | |
InChI |
InChI=1S/C15H11N3O/c19-11-9-14(12-5-1-3-7-16-12)18-15(10-11)13-6-2-4-8-17-13/h1-10H,(H,18,19) | |
Source | PubChem | |
URL | https://pubchem.ncbi.nlm.nih.gov | |
Description | Data deposited in or computed by PubChem | |
InChI Key |
HRORSVNZQWCZTD-UHFFFAOYSA-N | |
Source | PubChem | |
URL | https://pubchem.ncbi.nlm.nih.gov | |
Description | Data deposited in or computed by PubChem | |
Canonical SMILES |
C1=CC=NC(=C1)C2=CC(=O)C=C(N2)C3=CC=CC=N3 | |
Source | PubChem | |
URL | https://pubchem.ncbi.nlm.nih.gov | |
Description | Data deposited in or computed by PubChem | |
Molecular Formula |
C15H11N3O | |
Source | PubChem | |
URL | https://pubchem.ncbi.nlm.nih.gov | |
Description | Data deposited in or computed by PubChem | |
Molecular Weight |
249.27 g/mol | |
Source | PubChem | |
URL | https://pubchem.ncbi.nlm.nih.gov | |
Description | Data deposited in or computed by PubChem | |
CAS No. |
128143-88-4 | |
Record name | 2,6-Bis(2-pyridyl)-4(1H)-pyridone | |
Source | European Chemicals Agency (ECHA) | |
URL | https://echa.europa.eu/information-on-chemicals | |
Description | The European Chemicals Agency (ECHA) is an agency of the European Union which is the driving force among regulatory authorities in implementing the EU's groundbreaking chemicals legislation for the benefit of human health and the environment as well as for innovation and competitiveness. | |
Explanation | Use of the information, documents and data from the ECHA website is subject to the terms and conditions of this Legal Notice, and subject to other binding limitations provided for under applicable law, the information, documents and data made available on the ECHA website may be reproduced, distributed and/or used, totally or in part, for non-commercial purposes provided that ECHA is acknowledged as the source: "Source: European Chemicals Agency, http://echa.europa.eu/". Such acknowledgement must be included in each copy of the material. ECHA permits and encourages organisations and individuals to create links to the ECHA website under the following cumulative conditions: Links can only be made to webpages that provide a link to the Legal Notice page. | |
Synthesis routes and methods I
Procedure details
Synthesis routes and methods II
Procedure details
Synthesis routes and methods III
Procedure details
Retrosynthesis Analysis
AI-Powered Synthesis Planning: Our tool employs the Template_relevance Pistachio, Template_relevance Bkms_metabolic, Template_relevance Pistachio_ringbreaker, Template_relevance Reaxys, Template_relevance Reaxys_biocatalysis model, leveraging a vast database of chemical reactions to predict feasible synthetic routes.
One-Step Synthesis Focus: Specifically designed for one-step synthesis, it provides concise and direct routes for your target compounds, streamlining the synthesis process.
Accurate Predictions: Utilizing the extensive PISTACHIO, BKMS_METABOLIC, PISTACHIO_RINGBREAKER, REAXYS, REAXYS_BIOCATALYSIS database, our tool offers high-accuracy predictions, reflecting the latest in chemical research and data.
Strategy Settings
Precursor scoring | Relevance Heuristic |
---|---|
Min. plausibility | 0.01 |
Model | Template_relevance |
Template Set | Pistachio/Bkms_metabolic/Pistachio_ringbreaker/Reaxys/Reaxys_biocatalysis |
Top-N result to add to graph | 6 |
Feasible Synthetic Routes
Disclaimer and Information on In-Vitro Research Products
Please be aware that all articles and product information presented on BenchChem are intended solely for informational purposes. The products available for purchase on BenchChem are specifically designed for in-vitro studies, which are conducted outside of living organisms. In-vitro studies, derived from the Latin term "in glass," involve experiments performed in controlled laboratory settings using cells or tissues. It is important to note that these products are not categorized as medicines or drugs, and they have not received approval from the FDA for the prevention, treatment, or cure of any medical condition, ailment, or disease. We must emphasize that any form of bodily introduction of these products into humans or animals is strictly prohibited by law. It is essential to adhere to these guidelines to ensure compliance with legal and ethical standards in research and experimentation.