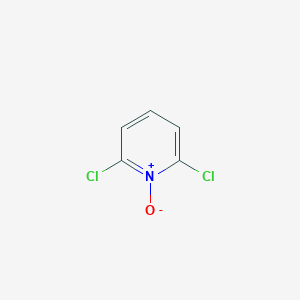
2,6-Dichloropyridine N-oxide
Overview
Description
2,6-Dichloropyridine N-oxide is an organic compound with the molecular formula C5H3Cl2NO. It is a white to almost white crystalline solid that is primarily used in organic synthesis. This compound is known for its role as an intermediate in the production of various pharmaceuticals and agrochemicals .
Biochemical Analysis
Biochemical Properties
2,6-Dichloropyridine 1-oxide plays a significant role in biochemical reactions, particularly as an intermediate in the synthesis of various pharmaceuticals and agrochemicals. It interacts with several enzymes and proteins, influencing their activity and function. For instance, it has been observed to interact with cytochrome P450 enzymes, which are involved in the metabolism of many drugs and xenobiotics. The nature of these interactions often involves the inhibition or activation of enzyme activity, depending on the specific enzyme and the context of the reaction .
Cellular Effects
The effects of 2,6-Dichloropyridine 1-oxide on various types of cells and cellular processes are profound. It influences cell function by affecting cell signaling pathways, gene expression, and cellular metabolism. For example, it has been shown to modulate the activity of certain transcription factors, leading to changes in gene expression profiles. Additionally, 2,6-Dichloropyridine 1-oxide can impact cellular metabolism by altering the activity of metabolic enzymes, thereby affecting the overall metabolic flux within the cell .
Molecular Mechanism
At the molecular level, 2,6-Dichloropyridine 1-oxide exerts its effects through specific binding interactions with biomolecules. It can bind to the active sites of enzymes, either inhibiting or activating their function. This binding often involves interactions with key amino acid residues within the enzyme’s active site. Furthermore, 2,6-Dichloropyridine 1-oxide can influence gene expression by interacting with transcription factors and other regulatory proteins, leading to changes in the transcriptional activity of target genes .
Temporal Effects in Laboratory Settings
In laboratory settings, the effects of 2,6-Dichloropyridine 1-oxide can change over time. The compound’s stability and degradation are important factors that influence its long-term effects on cellular function. Studies have shown that 2,6-Dichloropyridine 1-oxide is relatively stable under standard laboratory conditions, but it can degrade over time, leading to a decrease in its activity. Long-term exposure to this compound in in vitro or in vivo studies has been associated with changes in cellular function, including alterations in cell proliferation and differentiation .
Dosage Effects in Animal Models
The effects of 2,6-Dichloropyridine 1-oxide vary with different dosages in animal models. At low doses, the compound may have minimal or beneficial effects, while at higher doses, it can exhibit toxic or adverse effects. For instance, studies in animal models have shown that high doses of 2,6-Dichloropyridine 1-oxide can lead to liver toxicity and other adverse effects. These threshold effects highlight the importance of careful dosage control in experimental settings .
Metabolic Pathways
2,6-Dichloropyridine 1-oxide is involved in several metabolic pathways, interacting with various enzymes and cofactors. It is metabolized primarily by cytochrome P450 enzymes, which catalyze its oxidation and subsequent breakdown. This metabolism can lead to the formation of reactive intermediates, which may further interact with cellular components, affecting metabolic flux and metabolite levels .
Transport and Distribution
Within cells and tissues, 2,6-Dichloropyridine 1-oxide is transported and distributed through specific transporters and binding proteins. These interactions influence its localization and accumulation within different cellular compartments. For example, the compound may be actively transported into the mitochondria or other organelles, where it can exert its biochemical effects. The distribution of 2,6-Dichloropyridine 1-oxide within tissues is also influenced by its physicochemical properties, such as its solubility and affinity for different cellular components .
Subcellular Localization
The subcellular localization of 2,6-Dichloropyridine 1-oxide is crucial for its activity and function. It can be directed to specific compartments or organelles through targeting signals or post-translational modifications. For instance, the compound may be localized to the nucleus, where it can interact with transcription factors and influence gene expression. Alternatively, it may be targeted to the endoplasmic reticulum or mitochondria, where it can affect protein folding and metabolic processes .
Preparation Methods
Synthetic Routes and Reaction Conditions: 2,6-Dichloropyridine N-oxide can be synthesized through the oxidation of 2,6-dichloropyridine. One common method involves the use of hydrogen peroxide in the presence of acetic acid as the oxidizing agent. The reaction is typically carried out under reflux conditions for several hours .
Industrial Production Methods: In industrial settings, the production of this compound often involves the chlorination of pyridine followed by oxidation. The chlorination step is performed using chlorine gas, and the resulting 2,6-dichloropyridine is then oxidized using hydrogen peroxide or other suitable oxidizing agents .
Chemical Reactions Analysis
Types of Reactions: 2,6-Dichloropyridine N-oxide undergoes various types of chemical reactions, including:
Oxidation: It can act as an oxidizing agent in organic synthesis, converting alcohols to ketones or aldehydes.
Substitution: The compound can participate in nucleophilic substitution reactions, where the chlorine atoms are replaced by other nucleophiles.
Common Reagents and Conditions:
Oxidation Reactions: Hydrogen peroxide, acetic acid, and other oxidizing agents are commonly used.
Substitution Reactions: Nucleophiles such as amines, thiols, and alkoxides are used under various conditions, often requiring a base to facilitate the reaction.
Major Products:
Oxidation: Ketones, aldehydes, and carboxylic acids.
Substitution: Various substituted pyridine derivatives.
Scientific Research Applications
2,6-Dichloropyridine N-oxide has a wide range of applications in scientific research:
Mechanism of Action
The mechanism of action of 2,6-dichloropyridine N-oxide involves its ability to act as an oxidizing agent. It can transfer oxygen atoms to various substrates, facilitating the conversion of alcohols to ketones or aldehydes. The molecular targets include hydroxyl groups in organic molecules, and the pathways involved are typically oxidation-reduction reactions .
Comparison with Similar Compounds
2-Chloropyridine: A halogenated derivative of pyridine used in the synthesis of pharmaceuticals and agrochemicals.
4-Chloropyridine N-oxide: Another pyridine N-oxide derivative with similar oxidizing properties.
2,6-Dichloro-4-nitropyridine N-oxide: A more complex derivative used in specialized organic synthesis.
Uniqueness: 2,6-Dichloropyridine N-oxide is unique due to its specific substitution pattern, which imparts distinct reactivity and selectivity in chemical reactions. Its ability to act as both an oxidizing agent and a substrate for nucleophilic substitution makes it a versatile compound in organic synthesis .
Properties
IUPAC Name |
2,6-dichloro-1-oxidopyridin-1-ium | |
---|---|---|
Source | PubChem | |
URL | https://pubchem.ncbi.nlm.nih.gov | |
Description | Data deposited in or computed by PubChem | |
InChI |
InChI=1S/C5H3Cl2NO/c6-4-2-1-3-5(7)8(4)9/h1-3H | |
Source | PubChem | |
URL | https://pubchem.ncbi.nlm.nih.gov | |
Description | Data deposited in or computed by PubChem | |
InChI Key |
RFOMGVDPYLWLOC-UHFFFAOYSA-N | |
Source | PubChem | |
URL | https://pubchem.ncbi.nlm.nih.gov | |
Description | Data deposited in or computed by PubChem | |
Canonical SMILES |
C1=CC(=[N+](C(=C1)Cl)[O-])Cl | |
Source | PubChem | |
URL | https://pubchem.ncbi.nlm.nih.gov | |
Description | Data deposited in or computed by PubChem | |
Molecular Formula |
C5H3Cl2NO | |
Source | PubChem | |
URL | https://pubchem.ncbi.nlm.nih.gov | |
Description | Data deposited in or computed by PubChem | |
DSSTOX Substance ID |
DTXSID50355830 | |
Record name | 2,6-Dichloropyridine N-oxide | |
Source | EPA DSSTox | |
URL | https://comptox.epa.gov/dashboard/DTXSID50355830 | |
Description | DSSTox provides a high quality public chemistry resource for supporting improved predictive toxicology. | |
Molecular Weight |
163.99 g/mol | |
Source | PubChem | |
URL | https://pubchem.ncbi.nlm.nih.gov | |
Description | Data deposited in or computed by PubChem | |
CAS No. |
2587-00-0 | |
Record name | 2587-00-0 | |
Source | DTP/NCI | |
URL | https://dtp.cancer.gov/dtpstandard/servlet/dwindex?searchtype=NSC&outputformat=html&searchlist=136569 | |
Description | The NCI Development Therapeutics Program (DTP) provides services and resources to the academic and private-sector research communities worldwide to facilitate the discovery and development of new cancer therapeutic agents. | |
Explanation | Unless otherwise indicated, all text within NCI products is free of copyright and may be reused without our permission. Credit the National Cancer Institute as the source. | |
Record name | 2,6-Dichloropyridine N-oxide | |
Source | EPA DSSTox | |
URL | https://comptox.epa.gov/dashboard/DTXSID50355830 | |
Description | DSSTox provides a high quality public chemistry resource for supporting improved predictive toxicology. | |
Record name | 2,6-Dichloropyridine N-Oxide | |
Source | European Chemicals Agency (ECHA) | |
URL | https://echa.europa.eu/information-on-chemicals | |
Description | The European Chemicals Agency (ECHA) is an agency of the European Union which is the driving force among regulatory authorities in implementing the EU's groundbreaking chemicals legislation for the benefit of human health and the environment as well as for innovation and competitiveness. | |
Explanation | Use of the information, documents and data from the ECHA website is subject to the terms and conditions of this Legal Notice, and subject to other binding limitations provided for under applicable law, the information, documents and data made available on the ECHA website may be reproduced, distributed and/or used, totally or in part, for non-commercial purposes provided that ECHA is acknowledged as the source: "Source: European Chemicals Agency, http://echa.europa.eu/". Such acknowledgement must be included in each copy of the material. ECHA permits and encourages organisations and individuals to create links to the ECHA website under the following cumulative conditions: Links can only be made to webpages that provide a link to the Legal Notice page. | |
Synthesis routes and methods I
Procedure details
Synthesis routes and methods II
Procedure details
Synthesis routes and methods III
Procedure details
Retrosynthesis Analysis
AI-Powered Synthesis Planning: Our tool employs the Template_relevance Pistachio, Template_relevance Bkms_metabolic, Template_relevance Pistachio_ringbreaker, Template_relevance Reaxys, Template_relevance Reaxys_biocatalysis model, leveraging a vast database of chemical reactions to predict feasible synthetic routes.
One-Step Synthesis Focus: Specifically designed for one-step synthesis, it provides concise and direct routes for your target compounds, streamlining the synthesis process.
Accurate Predictions: Utilizing the extensive PISTACHIO, BKMS_METABOLIC, PISTACHIO_RINGBREAKER, REAXYS, REAXYS_BIOCATALYSIS database, our tool offers high-accuracy predictions, reflecting the latest in chemical research and data.
Strategy Settings
Precursor scoring | Relevance Heuristic |
---|---|
Min. plausibility | 0.01 |
Model | Template_relevance |
Template Set | Pistachio/Bkms_metabolic/Pistachio_ringbreaker/Reaxys/Reaxys_biocatalysis |
Top-N result to add to graph | 6 |
Feasible Synthetic Routes
Q1: What is the primary application of 2,6-dichloropyridine N-oxide in scientific research?
A1: this compound is primarily employed as a stoichiometric oxidant in various organic reactions, particularly in the presence of transition metal catalysts like ruthenium porphyrins. [, , , , , , , , , , , , , , , , , , , , , , , , , , , ]
Q2: Which reactions are commonly catalyzed by ruthenium porphyrins using this compound as an oxidant?
A2: Ruthenium porphyrins, in conjunction with this compound, efficiently catalyze alkene epoxidation, alkane hydroxylation, and cyclopropanation reactions. [, , , , , , , , , , , , , , , , , , , , , , , , , , , ]
Q3: Can you elaborate on the role of this compound in these catalytic reactions?
A3: this compound acts as a source of oxygen atoms, effectively transferring an oxygen atom to the ruthenium porphyrin catalyst. This generates a highly reactive high-valent ruthenium-oxo species, which then interacts with the substrate (alkane, alkene, etc.) to facilitate the desired oxidation reaction. [, , , , , , , , , , , , , , , , , , , , , , , , , , , ]
Q4: What makes this compound a suitable oxidant in these reactions?
A4: The presence of electron-withdrawing chlorine atoms on the pyridine ring enhances the oxygen transfer capability of this compound, making it a highly effective oxidant in these catalytic systems. [, , , , , , , , , , , , , , , , , , , , , , , , , , , ]
Q5: Are there other metal complexes, besides ruthenium porphyrins, that utilize this compound as an oxidant?
A5: Yes, research indicates that this compound can act as an oxidant in reactions catalyzed by other metal complexes, including organoruthenium polyoxometalates and (salen)ruthenium(II) complexes. [, , ]
Q6: What are the advantages of using this compound as an oxidant in organic synthesis?
A6: Several advantages make this compound attractive:
Q7: Are there any limitations to using this compound in organic synthesis?
A7: While effective, some potential limitations exist:
Q8: What are the current research directions regarding this compound in catalysis?
A8: Current research focuses on:
Q9: What is the molecular formula and weight of this compound?
A9: The molecular formula is C5H3Cl2NO, and its molecular weight is 176.00 g/mol.
Q10: What spectroscopic techniques are typically used to characterize this compound?
A10: Common techniques include:
Q11: Are there any specific materials or conditions that this compound is incompatible with?
A11: While specific compatibility data might vary, it's generally incompatible with:
Q12: How do structural modifications of the pyridine ring in this compound affect its oxidizing ability?
A13: Introducing electron-withdrawing groups, such as chlorine atoms at the 2- and 6-positions, increases the electrophilicity of the oxygen atom in the N-oxide group, enhancing its oxidizing ability. Conversely, electron-donating groups would decrease its oxidizing potential. [, , ]
Disclaimer and Information on In-Vitro Research Products
Please be aware that all articles and product information presented on BenchChem are intended solely for informational purposes. The products available for purchase on BenchChem are specifically designed for in-vitro studies, which are conducted outside of living organisms. In-vitro studies, derived from the Latin term "in glass," involve experiments performed in controlled laboratory settings using cells or tissues. It is important to note that these products are not categorized as medicines or drugs, and they have not received approval from the FDA for the prevention, treatment, or cure of any medical condition, ailment, or disease. We must emphasize that any form of bodily introduction of these products into humans or animals is strictly prohibited by law. It is essential to adhere to these guidelines to ensure compliance with legal and ethical standards in research and experimentation.