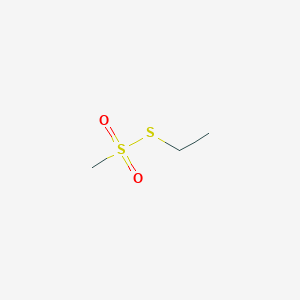
Ethyl Methanethiosulfonate
Overview
Description
1,2-Didecanoyl-sn-glycerol (CAS 60514-49-0) is a diacylglycerol (DAG) analog. It contains decanoic acid (a 10-carbon fatty acid) at both the sn-1 and sn-2 positions of the glycerol backbone. DAGs play a crucial role as second messengers in cellular signaling pathways, including activation of protein kinase C (PKC) . Although the specific biological activities of 1,2-Didecanoyl-sn-glycerol have not been extensively characterized, it is expected to behave similarly to 1,2-Dioctanoyl-sn-glycerol (Item No. 62225) .
Mechanism of Action
Target of Action
Ethyl Methanethiosulfonate primarily targets cysteine residues in proteins . It reacts with the thiol group (-SH) of cysteine, leading to the formation of mixed disulfides . This reaction is particularly important in the study of protein structure and function .
Mode of Action
The compound interacts with its targets by alkylating the guanine bases in DNA, forming an abnormal base called O6-ethylguanine . During DNA replication, DNA polymerases often place thymine, instead of cytosine, opposite this abnormal base . This leads to G:C to A:T transitions, which are a type of point mutation .
Biochemical Pathways
The primary biochemical pathway affected by this compound is DNA replication . The point mutations induced by the compound can lead to changes in the protein-coding sequence of genes, potentially altering their function. This is why this compound is commonly used in genetic research to induce mutations for study .
Result of Action
The primary result of this compound’s action is the induction of point mutations in DNA . These mutations can lead to changes in the amino acid sequence of proteins, potentially altering their structure and function. In a research context, this allows scientists to study the effects of these mutations.
Action Environment
The action, efficacy, and stability of this compound can be influenced by various environmental factors. For example, the pH and temperature of the environment can affect the rate of the alkylation reaction . Additionally, the presence of other reactive species can potentially interfere with the compound’s action.
Biochemical Analysis
Biochemical Properties
Ethyl Methanethiosulfonate is known to react specifically and rapidly with thiols to form mixed disulfides . This property makes it a powerful tool to probe the structures and functions of proteins, particularly membrane proteins such as ion channels .
Cellular Effects
This compound has been found to have significant effects on cellular function. For example, it has been shown to enhance the binding of certain compounds to specific receptors in a pH-dependent manner, but decrease the uptake of other compounds . It also has been found to cause base-pair insertions or deletions as well as more extensive intragenic deletions .
Molecular Mechanism
The molecular mechanism of this compound involves alkylation of cellular, nucleophilic sites via a mixed SN1/SN2 reaction mechanism . While ethylation of DNA occurs principally at nitrogen positions in the bases, this compound is also able to produce significant levels of alkylation at oxygens such as the O6 of guanine and in the DNA phosphate groups .
Temporal Effects in Laboratory Settings
In laboratory settings, this compound has been shown to have different effects over time. For instance, it has been found to enhance the binding of certain compounds to specific receptors in a pH-dependent manner, but decrease the uptake of other compounds .
Metabolic Pathways
It is known that this compound reacts with thiols to form mixed disulfides , suggesting that it may interact with enzymes or cofactors that contain thiol groups.
Preparation Methods
1,2-Didecanoyl-sn-glycerol can be synthesized through various routes:
Chemical Synthesis: One common method involves esterification of glycerol with decanoic acid (or its derivative) using acid-catalyzed or base-catalyzed conditions.
Enzymatic Synthesis: Lipases can catalyze the acylation of glycerol with decanoic acid.
Industrial Production: The industrial production of 1,2-Didecanoyl-sn-glycerol may involve large-scale enzymatic or chemical processes.
Chemical Reactions Analysis
1,2-Didecanoyl-sn-glycerol can participate in several reactions:
Hydrolysis: Under acidic or enzymatic conditions, it can be hydrolyzed back to glycerol and decanoic acid.
Oxidation/Reduction: DAGs can undergo oxidation (e.g., via lipases) or reduction (e.g., via hydrogenation).
Substitution: Functional group substitutions can occur at the sn-1 or sn-2 positions.
Common Reagents: Lipases, acids, bases, and reducing agents.
Major Products: Hydrolysis yields glycerol and decanoic acid, while other reactions may produce modified DAGs.
Scientific Research Applications
1,2-Didecanoyl-sn-glycerol finds applications in:
Cell Signaling Studies: As a DAG analog, it helps elucidate PKC activation pathways.
Lipid Metabolism Research: Investigating lipid signaling and cellular responses.
Drug Development: Targeting PKC-related diseases (e.g., cancer, diabetes, cardiovascular disorders).
Comparison with Similar Compounds
1,2-Dioctanoyl-sn-glycerol: Similar to 1,2-Didecanoyl-sn-glycerol, but with octanoic acid at both positions.
Other DAGs: Explore related diacylglycerols with varying acyl chain lengths.
Properties
IUPAC Name |
1-methylsulfonylsulfanylethane | |
---|---|---|
Source | PubChem | |
URL | https://pubchem.ncbi.nlm.nih.gov | |
Description | Data deposited in or computed by PubChem | |
InChI |
InChI=1S/C3H8O2S2/c1-3-6-7(2,4)5/h3H2,1-2H3 | |
Source | PubChem | |
URL | https://pubchem.ncbi.nlm.nih.gov | |
Description | Data deposited in or computed by PubChem | |
InChI Key |
IZAYCFBWPSFFJI-UHFFFAOYSA-N | |
Source | PubChem | |
URL | https://pubchem.ncbi.nlm.nih.gov | |
Description | Data deposited in or computed by PubChem | |
Canonical SMILES |
CCSS(=O)(=O)C | |
Source | PubChem | |
URL | https://pubchem.ncbi.nlm.nih.gov | |
Description | Data deposited in or computed by PubChem | |
Molecular Formula |
C3H8O2S2 | |
Source | PubChem | |
URL | https://pubchem.ncbi.nlm.nih.gov | |
Description | Data deposited in or computed by PubChem | |
DSSTOX Substance ID |
DTXSID40542530 | |
Record name | S-Ethyl methanesulfonothioate | |
Source | EPA DSSTox | |
URL | https://comptox.epa.gov/dashboard/DTXSID40542530 | |
Description | DSSTox provides a high quality public chemistry resource for supporting improved predictive toxicology. | |
Molecular Weight |
140.23 g/mol | |
Source | PubChem | |
URL | https://pubchem.ncbi.nlm.nih.gov | |
Description | Data deposited in or computed by PubChem | |
CAS No. |
2043-76-7 | |
Record name | S-Ethyl methanesulfonothioate | |
Source | CAS Common Chemistry | |
URL | https://commonchemistry.cas.org/detail?cas_rn=2043-76-7 | |
Description | CAS Common Chemistry is an open community resource for accessing chemical information. Nearly 500,000 chemical substances from CAS REGISTRY cover areas of community interest, including common and frequently regulated chemicals, and those relevant to high school and undergraduate chemistry classes. This chemical information, curated by our expert scientists, is provided in alignment with our mission as a division of the American Chemical Society. | |
Explanation | The data from CAS Common Chemistry is provided under a CC-BY-NC 4.0 license, unless otherwise stated. | |
Record name | S-Ethyl methanesulfonothioate | |
Source | EPA DSSTox | |
URL | https://comptox.epa.gov/dashboard/DTXSID40542530 | |
Description | DSSTox provides a high quality public chemistry resource for supporting improved predictive toxicology. | |
Retrosynthesis Analysis
AI-Powered Synthesis Planning: Our tool employs the Template_relevance Pistachio, Template_relevance Bkms_metabolic, Template_relevance Pistachio_ringbreaker, Template_relevance Reaxys, Template_relevance Reaxys_biocatalysis model, leveraging a vast database of chemical reactions to predict feasible synthetic routes.
One-Step Synthesis Focus: Specifically designed for one-step synthesis, it provides concise and direct routes for your target compounds, streamlining the synthesis process.
Accurate Predictions: Utilizing the extensive PISTACHIO, BKMS_METABOLIC, PISTACHIO_RINGBREAKER, REAXYS, REAXYS_BIOCATALYSIS database, our tool offers high-accuracy predictions, reflecting the latest in chemical research and data.
Strategy Settings
Precursor scoring | Relevance Heuristic |
---|---|
Min. plausibility | 0.01 |
Model | Template_relevance |
Template Set | Pistachio/Bkms_metabolic/Pistachio_ringbreaker/Reaxys/Reaxys_biocatalysis |
Top-N result to add to graph | 6 |
Feasible Synthetic Routes
Disclaimer and Information on In-Vitro Research Products
Please be aware that all articles and product information presented on BenchChem are intended solely for informational purposes. The products available for purchase on BenchChem are specifically designed for in-vitro studies, which are conducted outside of living organisms. In-vitro studies, derived from the Latin term "in glass," involve experiments performed in controlled laboratory settings using cells or tissues. It is important to note that these products are not categorized as medicines or drugs, and they have not received approval from the FDA for the prevention, treatment, or cure of any medical condition, ailment, or disease. We must emphasize that any form of bodily introduction of these products into humans or animals is strictly prohibited by law. It is essential to adhere to these guidelines to ensure compliance with legal and ethical standards in research and experimentation.