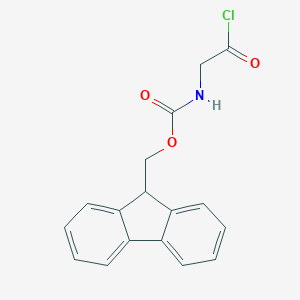
Fmoc-Gly-Cl
Overview
Description
9-Fluorenylmethyloxycarbonyl glycine chloride: (Fmoc-Gly-Cl) is a derivative of glycine where the amino group is protected by the 9-fluorenylmethyloxycarbonyl group. This compound is widely used in peptide synthesis, particularly in the solid-phase peptide synthesis (SPPS) method, due to its stability and ease of removal under basic conditions .
Mechanism of Action
Target of Action
The primary target of Fmoc-Gly-Cl, also known as fluorenylmethyloxycarbonyl chloride, is the amine group of amino acids and peptides . The Fmoc group serves as a protecting group for the amine, preventing it from reacting with other substances during peptide synthesis .
Mode of Action
This compound interacts with its targets through a process known as Fmoc protection . In this process, the Fmoc group is introduced by reacting the amine with Fmoc-Cl . This reaction results in the formation of a carbamate , effectively protecting the amine group .
Biochemical Pathways
Instead, it facilitates the synthesis of peptides , including ones of significant size and complexity . The Fmoc group can be removed by base, typically piperidine, which forms a stable adduct with the dibenzofulvene byproduct, preventing it from reacting with the substrate .
Pharmacokinetics
The fmoc group is rapidly removed by base, and this deprotection is a crucial step in solid-phase peptide synthesis .
Result of Action
The primary result of this compound’s action is the protection of the amine group during peptide synthesis . This protection allows for the successful synthesis of peptides without unwanted side reactions . After the peptide synthesis is complete, the Fmoc group can be removed, leaving the desired peptide product .
Action Environment
The action of this compound is influenced by several environmental factors. For instance, the reaction with the amine group is typically carried out in an organic solvent . The removal of the Fmoc group, on the other hand, requires a basic environment . The choice of solvent and the pH of the environment can significantly influence the efficiency of the Fmoc protection and deprotection processes .
Biochemical Analysis
Biochemical Properties
Fmoc-Gly-Cl plays a crucial role in biochemical reactions, particularly in the formation of peptides . It interacts with various biomolecules, primarily amines, where the Fmoc group can be introduced by reacting the amine with Fmoc-Cl . This interaction is fundamental in the process of peptide synthesis .
Cellular Effects
The effects of this compound on cells are primarily related to its role in peptide synthesis . By protecting amines during the synthesis process, this compound influences cell function, including impacts on cell signaling pathways, gene expression, and cellular metabolism .
Molecular Mechanism
The molecular mechanism of this compound involves its role as a protecting group for amines . It exerts its effects at the molecular level through binding interactions with amines, facilitating peptide synthesis . The Fmoc group is rapidly removed by base, allowing for the continuation of the peptide synthesis process .
Temporal Effects in Laboratory Settings
In laboratory settings, the effects of this compound can change over time. The Fmoc group is stable and resistant to treatment with trifluoroacetic acid (TFA) and hydrogen bromide/acetic acid . This stability ensures the effectiveness of this compound in peptide synthesis over time .
Metabolic Pathways
This compound is involved in the metabolic pathway of peptide synthesis . It interacts with amines and other enzymes during this process
Transport and Distribution
The transport and distribution of this compound within cells and tissues are closely tied to its role in peptide synthesis
Subcellular Localization
Given its role in peptide synthesis, it is likely to be found in areas of the cell where this process occurs
Preparation Methods
Synthetic Routes and Reaction Conditions: The preparation of 9-fluorenylmethyloxycarbonyl glycine chloride typically involves the reaction of glycine with 9-fluorenylmethyloxycarbonyl chloride (Fmoc-Cl). The reaction is carried out under Schotten-Baumann conditions, which involve the use of a base such as sodium bicarbonate in a solvent like dioxane or dimethylformamide (DMF) . The reaction proceeds as follows:
- Dissolve glycine in an aqueous solution of sodium bicarbonate.
- Add 9-fluorenylmethyloxycarbonyl chloride to the solution.
- Stir the mixture at room temperature until the reaction is complete.
- Extract the product with an organic solvent such as ethyl acetate.
- Purify the product by recrystallization or chromatography.
Industrial Production Methods: In industrial settings, the production of 9-fluorenylmethyloxycarbonyl glycine chloride follows similar principles but on a larger scale. The process involves the use of automated reactors and continuous flow systems to ensure consistent quality and yield. The reaction conditions are optimized to minimize by-products and maximize the efficiency of the process .
Chemical Reactions Analysis
Types of Reactions: 9-Fluorenylmethyloxycarbonyl glycine chloride primarily undergoes substitution reactions, particularly nucleophilic substitution, where the 9-fluorenylmethyloxycarbonyl group is introduced or removed.
Common Reagents and Conditions:
Introduction of 9-fluorenylmethyloxycarbonyl Group: This is typically done using 9-fluorenylmethyloxycarbonyl chloride in the presence of a base such as sodium bicarbonate or pyridine.
Removal of 9-fluorenylmethyloxycarbonyl Group: The 9-fluorenylmethyloxycarbonyl group is removed using a base such as piperidine in dimethylformamide (DMF).
Major Products Formed:
Introduction Reaction: The major product is 9-fluorenylmethyloxycarbonyl glycine chloride.
Removal Reaction: The major products are glycine and dibenzofulvene.
Scientific Research Applications
9-Fluorenylmethyloxycarbonyl glycine chloride is extensively used in scientific research, particularly in the fields of chemistry, biology, and medicine.
Chemistry:
Peptide Synthesis: It is a key reagent in the solid-phase peptide synthesis (SPPS) method, allowing for the efficient and selective protection of amino groups.
Biology:
Protein Engineering: It is used in the synthesis of peptides and proteins for studying protein structure and function.
Medicine:
Drug Development: It is used in the synthesis of peptide-based drugs and therapeutic agents.
Industry:
Comparison with Similar Compounds
9-Fluorenylmethyloxycarbonyl glycine chloride is unique among amino acid derivatives due to its stability and ease of removal under basic conditions. Similar compounds include:
tert-Butyloxycarbonyl glycine chloride (Boc-Gly-Cl): This compound uses the tert-butyloxycarbonyl group for protection, which is removed under acidic conditions.
Carbobenzoxy glycine chloride (Cbz-Gly-Cl): This compound uses the carbobenzoxy group for protection, which is also removed under acidic conditions.
The uniqueness of 9-fluorenylmethyloxycarbonyl glycine chloride lies in its base-labile protection, which allows for selective deprotection without affecting other acid-labile groups .
Properties
IUPAC Name |
9H-fluoren-9-ylmethyl N-(2-chloro-2-oxoethyl)carbamate | |
---|---|---|
Source | PubChem | |
URL | https://pubchem.ncbi.nlm.nih.gov | |
Description | Data deposited in or computed by PubChem | |
InChI |
InChI=1S/C17H14ClNO3/c18-16(20)9-19-17(21)22-10-15-13-7-3-1-5-11(13)12-6-2-4-8-14(12)15/h1-8,15H,9-10H2,(H,19,21) | |
Source | PubChem | |
URL | https://pubchem.ncbi.nlm.nih.gov | |
Description | Data deposited in or computed by PubChem | |
InChI Key |
HFWFBOCOXPEKBV-UHFFFAOYSA-N | |
Source | PubChem | |
URL | https://pubchem.ncbi.nlm.nih.gov | |
Description | Data deposited in or computed by PubChem | |
Canonical SMILES |
C1=CC=C2C(=C1)C(C3=CC=CC=C32)COC(=O)NCC(=O)Cl | |
Source | PubChem | |
URL | https://pubchem.ncbi.nlm.nih.gov | |
Description | Data deposited in or computed by PubChem | |
Molecular Formula |
C17H14ClNO3 | |
Source | PubChem | |
URL | https://pubchem.ncbi.nlm.nih.gov | |
Description | Data deposited in or computed by PubChem | |
DSSTOX Substance ID |
DTXSID90471922 | |
Record name | Fmoc-Gly-Cl | |
Source | EPA DSSTox | |
URL | https://comptox.epa.gov/dashboard/DTXSID90471922 | |
Description | DSSTox provides a high quality public chemistry resource for supporting improved predictive toxicology. | |
Molecular Weight |
315.7 g/mol | |
Source | PubChem | |
URL | https://pubchem.ncbi.nlm.nih.gov | |
Description | Data deposited in or computed by PubChem | |
CAS No. |
103321-49-9 | |
Record name | Fmoc-Gly-Cl | |
Source | EPA DSSTox | |
URL | https://comptox.epa.gov/dashboard/DTXSID90471922 | |
Description | DSSTox provides a high quality public chemistry resource for supporting improved predictive toxicology. | |
Record name | Fmoc-Gly-Cl | |
Source | European Chemicals Agency (ECHA) | |
URL | https://echa.europa.eu/information-on-chemicals | |
Description | The European Chemicals Agency (ECHA) is an agency of the European Union which is the driving force among regulatory authorities in implementing the EU's groundbreaking chemicals legislation for the benefit of human health and the environment as well as for innovation and competitiveness. | |
Explanation | Use of the information, documents and data from the ECHA website is subject to the terms and conditions of this Legal Notice, and subject to other binding limitations provided for under applicable law, the information, documents and data made available on the ECHA website may be reproduced, distributed and/or used, totally or in part, for non-commercial purposes provided that ECHA is acknowledged as the source: "Source: European Chemicals Agency, http://echa.europa.eu/". Such acknowledgement must be included in each copy of the material. ECHA permits and encourages organisations and individuals to create links to the ECHA website under the following cumulative conditions: Links can only be made to webpages that provide a link to the Legal Notice page. | |
Retrosynthesis Analysis
AI-Powered Synthesis Planning: Our tool employs the Template_relevance Pistachio, Template_relevance Bkms_metabolic, Template_relevance Pistachio_ringbreaker, Template_relevance Reaxys, Template_relevance Reaxys_biocatalysis model, leveraging a vast database of chemical reactions to predict feasible synthetic routes.
One-Step Synthesis Focus: Specifically designed for one-step synthesis, it provides concise and direct routes for your target compounds, streamlining the synthesis process.
Accurate Predictions: Utilizing the extensive PISTACHIO, BKMS_METABOLIC, PISTACHIO_RINGBREAKER, REAXYS, REAXYS_BIOCATALYSIS database, our tool offers high-accuracy predictions, reflecting the latest in chemical research and data.
Strategy Settings
Precursor scoring | Relevance Heuristic |
---|---|
Min. plausibility | 0.01 |
Model | Template_relevance |
Template Set | Pistachio/Bkms_metabolic/Pistachio_ringbreaker/Reaxys/Reaxys_biocatalysis |
Top-N result to add to graph | 6 |
Feasible Synthetic Routes
Disclaimer and Information on In-Vitro Research Products
Please be aware that all articles and product information presented on BenchChem are intended solely for informational purposes. The products available for purchase on BenchChem are specifically designed for in-vitro studies, which are conducted outside of living organisms. In-vitro studies, derived from the Latin term "in glass," involve experiments performed in controlled laboratory settings using cells or tissues. It is important to note that these products are not categorized as medicines or drugs, and they have not received approval from the FDA for the prevention, treatment, or cure of any medical condition, ailment, or disease. We must emphasize that any form of bodily introduction of these products into humans or animals is strictly prohibited by law. It is essential to adhere to these guidelines to ensure compliance with legal and ethical standards in research and experimentation.