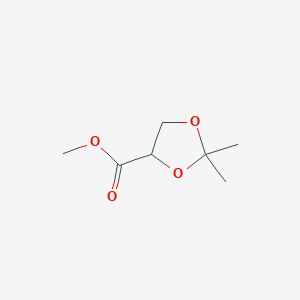
Methyl 2,2-dimethyl-1,3-dioxolane-4-carboxylate
Overview
Description
Synthesis Analysis
The synthesis of Methyl 2,2-dimethyl-1,3-dioxolane-4-carboxylate involves several key steps that ensure the formation of this compound with high purity and yield. A notable approach is the protection of dihydroxy compounds in the dioxolane form, leading to the formation of this compound as a protected intermediate (Mori, Hirao, & Nakahama, 1994).
Scientific Research Applications
Polymerization and Hydrogenation Applications : Shostakovskii et al. (1966) demonstrated the synthesis and characterization of 2,2-dialkyl-4-(vinyloxymethyl)-1,3-dioxolanes, which have potential applications in polymerization and hydrogenation processes (Shostakovskii et al., 1966).
Model Compounds in Borate Monoester Studies : Duin et al. (2010) reported that 1,3-dioxolane and its methyl-substituted derivatives serve as model compounds for borate monoesters of vicinal diols, with their conformations and pseudorotations aligning well with experimental values (Duin et al., 2010).
Synthesis of Chiral Diamines : Shainyan et al. (2002) synthesized new chiral diamines by combining 2,2-dimethyl-4,5-bis(diphenylaminomethyl)-1,3-dioxolane, highlighting their unique chemistry (Shainyan et al., 2002).
Intermediates in Synthesis of Chrysanthemic Acid : Baudoux et al. (1998) discussed how three compounds, including 2,2-dimethyl-1,3-dioxolane derivatives, are intermediates in the synthesis of chrysanthemic acid, offering insights into their chemistry and potential applications (Baudoux et al., 1998).
UV-Induced Crosslinking in Vinyl Monomers : D'alelio and Huemmer (1967) found that the 1,3-dioxolane group in vinyl monomers acts as an internal ultraviolet sensitizer, leading to crosslinking behavior when exposed to UV light (D'alelio & Huemmer, 1967).
Photolysis in Aqueous Media : Nikolaev et al. (1985) reported that photolysis of 5-diazo-2,2-dimethyl-4,6-dioxo-1,3-dioxane in aqueous media leads to the Wolff rearrangement, resulting in stable compounds (Nikolaev et al., 1985).
Antifungal and Antibacterial Properties : Begum et al. (2019) synthesized novel 1,3-dioxolane amide derivatives that exhibited promising antifungal and antibacterial activities against various strains (Begum et al., 2019).
Applications in Organic Synthesis : Kametani and Sumi (1972) noted that di- and trialkyl substituted 1,3-dioxolanes have distinct structural features, showing potential for applications in organic synthesis (Kametani & Sumi, 1972).
Synthesis of Optically Active Compounds : Yakura et al. (1999) described the dirhodium(II)-catalyzed C-H insertion reactions of 5,6-dioxygenated 2-diazo-3-oxohexanoates, which can be used in the synthesis of optically active, highly functionalized cyclopentane (Yakura et al., 1999).
Vapor Pressure Data and Equilibrium Studies : Chopade et al. (2003) studied the vapor-liquid-liquid equilibrium and vapor pressure data for systems involving 2-methyl-1,3-dioxolane and 2,4-dimethyl-1,3-dioxolane with water, providing valuable data for thermodynamic analyses (Chopade et al., 2003).
Safety and Hazards
This compound is classified as a combustible liquid. Precautionary measures include avoiding heat, sparks, open flames, and hot surfaces. Protective gloves, eye protection, and face protection should be worn when handling this compound. In case of fire, dry sand, dry chemical, or alcohol-resistant foam should be used to extinguish it .
Mechanism of Action
Target of Action
Methyl 2,2-dimethyl-1,3-dioxolane-4-carboxylate is a chiral building block . It is primarily used in the synthesis of other compounds, rather than having a specific biological target of its own .
Mode of Action
This compound is used as a starting material in chemical reactions. For instance, it can be used to make the key tetrahydrofuran subunit of (−)-gymnodimine, a marine algal toxin . It can also be used to prepare an enedione by reacting with dimethyl methylphosphonate, BuLi, and phenylglyoxal, which in turn is used to synthesize cyclopentenone derivatives .
Biochemical Pathways
As a building block, this compound is involved in the synthesis of various biochemicals. For example, it is used in the synthesis of enantiopure (S)-4,5-dihydroxy-2,3-pentanedione (DPD), which is the precursor of autoinducer (AI)-2, a proposed signal for bacterial interspecies communication .
Result of Action
The result of the action of this compound is the formation of other compounds. For example, it can be used to synthesize (2 R)- [1- 2 H 2 ]-glycerol, a probe to study the stereochemistry of glycerol metabolism in Streptomyces cattleya .
Action Environment
The action of this compound is influenced by the conditions of the chemical reactions in which it is used. Factors such as temperature, pH, and the presence of other reactants or catalysts can affect the yield and selectivity of the reactions .
properties
IUPAC Name |
methyl 2,2-dimethyl-1,3-dioxolane-4-carboxylate | |
---|---|---|
Source | PubChem | |
URL | https://pubchem.ncbi.nlm.nih.gov | |
Description | Data deposited in or computed by PubChem | |
InChI |
InChI=1S/C7H12O4/c1-7(2)10-4-5(11-7)6(8)9-3/h5H,4H2,1-3H3 | |
Source | PubChem | |
URL | https://pubchem.ncbi.nlm.nih.gov | |
Description | Data deposited in or computed by PubChem | |
InChI Key |
DOWWCCDWPKGNGX-UHFFFAOYSA-N | |
Source | PubChem | |
URL | https://pubchem.ncbi.nlm.nih.gov | |
Description | Data deposited in or computed by PubChem | |
Canonical SMILES |
CC1(OCC(O1)C(=O)OC)C | |
Source | PubChem | |
URL | https://pubchem.ncbi.nlm.nih.gov | |
Description | Data deposited in or computed by PubChem | |
Molecular Formula |
C7H12O4 | |
Source | PubChem | |
URL | https://pubchem.ncbi.nlm.nih.gov | |
Description | Data deposited in or computed by PubChem | |
Molecular Weight |
160.17 g/mol | |
Source | PubChem | |
URL | https://pubchem.ncbi.nlm.nih.gov | |
Description | Data deposited in or computed by PubChem | |
Retrosynthesis Analysis
AI-Powered Synthesis Planning: Our tool employs the Template_relevance Pistachio, Template_relevance Bkms_metabolic, Template_relevance Pistachio_ringbreaker, Template_relevance Reaxys, Template_relevance Reaxys_biocatalysis model, leveraging a vast database of chemical reactions to predict feasible synthetic routes.
One-Step Synthesis Focus: Specifically designed for one-step synthesis, it provides concise and direct routes for your target compounds, streamlining the synthesis process.
Accurate Predictions: Utilizing the extensive PISTACHIO, BKMS_METABOLIC, PISTACHIO_RINGBREAKER, REAXYS, REAXYS_BIOCATALYSIS database, our tool offers high-accuracy predictions, reflecting the latest in chemical research and data.
Strategy Settings
Precursor scoring | Relevance Heuristic |
---|---|
Min. plausibility | 0.01 |
Model | Template_relevance |
Template Set | Pistachio/Bkms_metabolic/Pistachio_ringbreaker/Reaxys/Reaxys_biocatalysis |
Top-N result to add to graph | 6 |
Feasible Synthetic Routes
Q & A
Q1: What are some synthetic applications of Methyl 2,2-dimethyl-1,3-dioxolane-4-carboxylate?
A1: this compound serves as a versatile building block in organic synthesis. For instance:
- Synthesis of Deuterated Metoprolol Enantiomers: It can be utilized as a starting material to synthesize enantiomers of metoprolol with specific deuterium substitutions. Specifically, the (4S)-Methyl 2,2-dimethyl-1,3-dioxolane-4-carboxylate enantiomer plays a key role in this process. []
- Synthesis of Pentenomycin I and Epipentenomycin I: The compound acts as a precursor in the synthesis of (±)-Pentenomycin I and (±)-epipentenomycin I. This synthetic route leverages the intramolecular acylation of α-sulfinyl carbanion derived from this compound, followed by pyrolysis. []
- Synthesis of (−)-Dihydromahubanolide B and (−)-Isodihydromahubanolide B: Starting from (−)-methyl 5-hydroxymethyl-2,2-dimethyl-1,3-dioxolane-4-carboxylate, which itself is readily derived from L-(+)-tartaric acid, researchers achieved the total synthesis of (−)-dihydromahubanolide B and (−)-isodihydromahubanolide B, compounds isolated from the Amazonian Lauraceae Licariamahuba (Samp.) Kosterm. []
Q2: Is there a specific enantiomer of this compound preferred in certain synthetic applications?
A: Yes, the stereochemistry of this compound can be crucial. For example, in the stereospecific synthesis of deuterated metoprolol enantiomers, both (4R)- and (4S)-Methyl 2,2-dimethyl-1,3-dioxolane-4-methanols are utilized as key chiral synthons. [] This highlights the significance of enantiomeric purity in achieving the desired stereochemistry in the final product.
Disclaimer and Information on In-Vitro Research Products
Please be aware that all articles and product information presented on BenchChem are intended solely for informational purposes. The products available for purchase on BenchChem are specifically designed for in-vitro studies, which are conducted outside of living organisms. In-vitro studies, derived from the Latin term "in glass," involve experiments performed in controlled laboratory settings using cells or tissues. It is important to note that these products are not categorized as medicines or drugs, and they have not received approval from the FDA for the prevention, treatment, or cure of any medical condition, ailment, or disease. We must emphasize that any form of bodily introduction of these products into humans or animals is strictly prohibited by law. It is essential to adhere to these guidelines to ensure compliance with legal and ethical standards in research and experimentation.