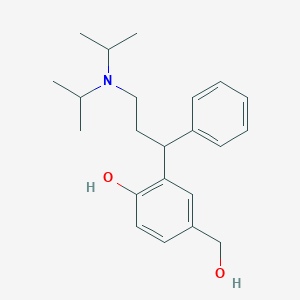
2-(3-(Diisopropylamino)-1-phenylpropyl)-4-(hydroxymethyl)phenol
Overview
Description
2-(3-(Diisopropylamino)-1-phenylpropyl)-4-(hydroxymethyl)phenol (IUPAC name: 2-[(1R)-3-(Diisopropylamino)-1-phenylpropyl]-4-(hydroxymethyl)phenol) is a chiral tertiary amine derivative with a molecular formula of C22H31NO2 and a molecular weight of 341.495 g/mol . It features a hydroxymethyl group at the 4-position of the phenolic ring and a diisopropylamino substituent at the 3-position of the propyl chain. This compound is pharmacologically significant as the active metabolite of Fesoterodine, a prodrug used to treat overactive bladder (OAB) by acting as a muscarinic receptor antagonist . Its stereochemistry (R-configuration) is critical for binding affinity and therapeutic efficacy .
Biological Activity
2-(3-(Diisopropylamino)-1-phenylpropyl)-4-(hydroxymethyl)phenol, commonly referred to as a derivative of Fesoterodine, is a compound with significant biological activity, particularly in the realm of pharmacology. This article explores its chemical properties, biological mechanisms, and potential therapeutic applications based on diverse research findings.
- Chemical Formula : C22H31NO2
- Molecular Weight : 341.49 g/mol
- CAS Number : 200801-70-3
- Molecular Structure : The compound features a diisopropylamino group and a hydroxymethyl phenol moiety, contributing to its pharmacological profile.
The biological activity of this compound is primarily linked to its role as a muscarinic receptor antagonist. It selectively inhibits the M3 muscarinic receptors, which are involved in various physiological processes, including smooth muscle contraction and glandular secretion.
Key Findings:
- Inhibition of Smooth Muscle Contraction : Research indicates that antagonism of M3 receptors can lead to decreased bladder contractions, making it potentially useful in treating overactive bladder conditions .
- Neuroprotective Effects : Some studies suggest that compounds with similar structures may exhibit neuroprotective properties by modulating neurotransmitter release and reducing oxidative stress .
Biological Activity Data
Case Studies
- Overactive Bladder Treatment : A clinical trial involving Fesoterodine (the parent compound) demonstrated improved bladder control and reduced urgency in patients with overactive bladder syndrome. The study highlighted the efficacy of muscarinic antagonists in managing symptoms effectively.
- Neuroprotection in Alzheimer's Disease Models : In vitro studies have indicated that derivatives of this compound may protect against amyloid-beta-induced toxicity, suggesting potential applications in neurodegenerative disorders .
Safety Profile
While the compound exhibits promising biological activity, safety assessments are crucial:
- Toxicity Studies : Preliminary data suggest low toxicity levels at therapeutic doses; however, further long-term studies are needed to establish a comprehensive safety profile.
- Regulatory Status : As a chemical entity under investigation, it is essential for ongoing research to comply with regulatory standards for pharmacological compounds.
Scientific Research Applications
Pharmaceutical Development
The primary application of 2-(3-(Diisopropylamino)-1-phenylpropyl)-4-(hydroxymethyl)phenol is in the development of medications for OAB. Its mechanism involves antagonizing muscarinic receptors, which helps reduce involuntary bladder contractions.
Case Study: Fesoterodine
Fesoterodine is a prodrug that is metabolized into the active form of tolterodine. Studies have shown that fesoterodine provides effective symptom relief for patients with OAB, demonstrating a favorable side effect profile compared to older anticholinergics. Clinical trials have indicated that fesoterodine significantly improves patient quality of life and reduces urinary frequency and urgency .
Synthesis and Derivative Research
Research into the synthesis of this compound has led to various derivatives with enhanced pharmacological properties. For instance, modifications to the hydroxymethyl group have been explored to improve solubility and bioavailability.
Synthetic Pathways
The synthesis of this compound often involves reactions such as acylation and alkylation, utilizing starting materials like isobutyryl chloride and triethylamine under controlled conditions .
Q & A
Basic Research Questions
Q. What analytical methods are recommended for structural confirmation of this compound?
Methodological Answer: Use a combination of nuclear magnetic resonance (NMR) spectroscopy (¹H and ¹³C) and high-performance liquid chromatography (HPLC) with a polar stationary phase (e.g., C18 column) and a mobile phase of methanol/sodium acetate buffer (65:35, pH 4.6) to resolve stereoisomers and confirm substituent positions . Mass spectrometry (MS) with electrospray ionization (ESI) can further validate molecular weight and fragmentation patterns.
Q. How can researchers assess the compound’s purity and stability under laboratory conditions?
Methodological Answer: Employ accelerated stability testing by exposing the compound to stressors (e.g., heat, light, humidity) and monitoring degradation via HPLC-UV. Use peak area normalization for quantitative purity assessment. For pH-dependent stability, conduct kinetic studies in buffered solutions (pH 3–9) to identify optimal storage conditions .
Q. What safety protocols are critical for handling this compound in laboratory settings?
Methodological Answer: Follow SDS guidelines, including the use of nitrile gloves, fume hoods, and sealed containers to minimize inhalation/contact risks. Store at –20°C under inert gas (e.g., argon) to prevent oxidation. Waste disposal must comply with local regulations for amine-containing phenolics .
Advanced Research Questions
Q. How can experimental design address contradictions in environmental fate data for this compound?
Methodological Answer: Implement a tiered approach:
- Tier 1: Determine octanol-water partition coefficients (log Kow) via shake-flask methods to predict bioaccumulation potential.
- Tier 2: Conduct soil/water microcosm studies under controlled pH and temperature to quantify abiotic hydrolysis rates.
- Tier 3: Use LC-MS/MS to identify transformation products in biotic systems (e.g., microbial communities) . Discrepancies in degradation rates between studies may arise from variations in microbial activity or redox conditions, necessitating multivariate statistical validation .
Q. What methodologies resolve enantiomeric-specific bioactivity discrepancies in chiral synthesis?
Methodological Answer: Optimize enantioselective synthesis using chiral auxiliaries (e.g., Evans oxazolidinones) or asymmetric catalysis (e.g., BINAP-ruthenium complexes). Validate enantiomeric excess (ee) via chiral HPLC with cellulose-based columns. Compare bioactivity of (R)- and (S)-enantiomers in receptor-binding assays (e.g., radioligand displacement) to isolate stereochemical effects .
Q. How can computational models predict the compound’s interactions with biological targets?
Methodological Answer: Apply molecular docking (e.g., AutoDock Vina) to simulate binding to putative targets (e.g., G-protein-coupled receptors). Validate predictions with surface plasmon resonance (SPR) to measure binding kinetics (KD, kon, koff). Cross-reference with QSAR models to correlate substituent effects (e.g., diisopropylamino hydrophobicity) with activity .
Q. What strategies mitigate variability in cytotoxicity assays across cell lines?
Methodological Answer: Standardize assay conditions using ISO-certified cell lines (e.g., HepG2 for hepatic toxicity) and control for metabolic activity (e.g., MTT assay normalization). Employ high-content screening (HCS) to quantify subcellular effects (e.g., mitochondrial membrane potential). Use ANOVA to identify confounding factors (e.g., serum batch variability) .
Q. How can researchers optimize synthetic routes to improve yield and scalability?
Methodological Answer: Apply design of experiments (DoE) to evaluate reaction parameters (e.g., temperature, catalyst loading). Use microwave-assisted synthesis to reduce reaction times. Characterize intermediates via in-situ FTIR to monitor progress. Compare yields between Ullmann coupling and Buchwald-Hartwig amination for aryl-amine bond formation .
Q. Data Contradiction Analysis
Q. How should researchers interpret conflicting toxicity data between in vitro and in vivo models?
Methodological Answer: Perform physiologically based pharmacokinetic (PBPK) modeling to reconcile differences. For example, low in vivo bioavailability due to first-pass metabolism may explain reduced toxicity compared to in vitro IC50 values. Validate with portal vein cannulation studies in rodents to measure systemic exposure .
Q. What statistical approaches address variability in antioxidant activity measurements?
Methodological Answer: Use Bland-Altman analysis to assess agreement between methods (e.g., DPPH vs. ORAC assays). Control for auto-oxidation by including Trolox as a reference standard. Apply principal component analysis (PCA) to identify assay-specific biases (e.g., pH sensitivity) .
Comparison with Similar Compounds
Comparison with Structurally and Functionally Related Compounds
Structural Analogues
Tolterodine
- Structure : Differs by a methyl group at the 4-position instead of hydroxymethyl.
- Molecular Formula: C22H31NO2 (same as target compound but with methyl substitution).
- Pharmacology : Direct-acting antimuscarinic agent for OAB. Unlike the target compound, Tolterodine requires hepatic metabolism (via CYP2D6) to form its active 5-hydroxymethyl metabolite, which structurally matches the target compound .
(R)-5-Hydroxytolterodine
- Structure : Identical to the target compound (CAS: 207679-81-0) .
- Role : Active metabolite of Tolterodine. Demonstrates higher muscarinic receptor affinity than the parent drug due to the hydroxymethyl group .
(R)-4-(Hydroxymethyl)-2-(3-(isopropylamino)-1-phenylpropyl)phenol
- Structure: Replaces the diisopropylamino group with a mono-isopropylamino group.
- Molecular Weight : 299.41 g/mol (vs. 341.495 g/mol for the target compound) .
- Impact : Reduced steric bulk may alter receptor binding kinetics or metabolic stability.
Prodrug Derivatives
Fesoterodine Fumarate
- Structure : Prodrug of the target compound, featuring an isobutyrate ester at the hydroxymethyl group and a fumarate counterion.
- Molecular Formula: C26H37NO3·C4H4O4 (ester + salt form; molecular weight: 527.65 g/mol) .
- Pharmacology: Rapidly hydrolyzed by nonspecific esterases in vivo to release the target compound, bypassing CYP-dependent metabolism. This design improves oral bioavailability and provides consistent dosing across CYP2D6 phenotypes .
- Key Advantage : Prodrug strategy enhances lipophilicity, facilitating intestinal absorption .
Salts and Impurities
2-(3-(Diisopropylamino)-1-phenylpropyl)-4-(hydroxymethyl)phenol Hydrochloride
- Structure : Hydrochloride salt of the target compound.
- Molecular Formula: C22H32ClNO2 (molecular weight: 377.95 g/mol) .
- Use : Reference standard for impurity profiling in Fesoterodine manufacturing .
Tolterodine Tartrate
- Structure : Tartrate salt of Tolterodine.
- Molecular Formula: C22H31NO·C4H6O6 (molecular weight: 475.57 g/mol) .
- Regulatory Status : USP-defined purity (97.0–103.0%) ensures clinical safety and efficacy .
Pharmacokinetic and Pharmacodynamic Comparisons
Metabolic Pathways
Receptor Affinity and Potency
Preparation Methods
Synthetic Pathways and Reaction Mechanisms
Tolterodine-Based Synthesis (WO2005012227A2)
The most widely documented method involves tolterodine hydrobromide as the starting material. This four-step process includes benzylation , oxidation , reduction , and debenzylation (Figure 1) .
Benzylation of Tolterodine
Tolterodine hydrobromide undergoes benzylation using benzyl chloride in a mixed solvent system (methanol:acetone, 1:1) with potassium carbonate and sodium iodide as catalysts. The reaction proceeds via nucleophilic substitution at the phenolic oxygen, yielding [3-(2-benzyloxy-5-methyl-phenyl)-3-phenyl-propyl]-diisopropylamine (Formula III) . Optimal conditions include refluxing for 3.25 hours, achieving near-quantitative conversion (14.1 g product from 15.3 g starting material) .
Oxidation to Benzaldehyde Derivative
The benzylated intermediate is oxidized using copper sulfate and sodium persulfate in a biphasic acetonitrile-water system. This step converts the methyl group to a formyl moiety, forming 4-benzyloxy-3-(3-diisopropylamino-1-phenyl-propyl)-benzaldehyde (Formula IV) . The reaction requires refluxing at 78–80°C for 4 hours, with dichloromethane extraction yielding 9.94 g of product .
Reduction to Benzyl Alcohol
Sodium borohydride in methanol reduces the aldehyde to [4-benzyloxy-3-(3-diisopropylamino-1-phenyl-propyl)-phenyl]-methanol (Formula V). Refluxing for 3 hours followed by chloroform extraction provides 8 g of crude product .
Debenzylation via Hydrogenolysis
Catalytic hydrogenation (10% Pd/C, 40–50 psi H₂) removes the benzyl protecting group, yielding the final product. Post-reaction purification via sodium carbonate washes and solvent evaporation affords 2.5 g of 2-(3-diisopropylamino-1-phenylpropyl)-4-hydroxymethyl-phenol with >98% purity .
Chroman-2-One Derivative Route (WO2011141932A2)
An alternative pathway starts with (±)-6-bromo-4-phenylchroman-2-one, involving reduction , hydroxyl protection , amine coupling , and Grignard reactions .
Reduction and Protection
Lithium aluminum hydride reduces the chroman-2-one to (±)-4-bromo-2-(3-hydroxy-1-phenylpropyl)phenol , followed by benzyl protection using benzyl bromide/K₂CO₃ in acetone .
Grignard Reaction and Esterification
Ethyl bromide/Mg-mediated Grignard addition introduces the hydroxymethyl group. Subsequent esterification with isobutyryl chloride and final deprotection yields the target compound .
Comparative Analysis of Methodologies
The tolterodine route offers higher purity but lower yield due to multi-step extraction and hydrogenation. The chroman-2-one pathway, while longer, provides modular intermediates for structural analogs .
Optimization Strategies and Challenges
Solvent Selection
-
Polar aprotic solvents (e.g., acetonitrile) enhance oxidation efficiency but require stringent drying .
-
Methanol/water mixtures improve sodium borohydride stability during reduction .
Catalytic Hydrogenation
-
Pd/C loading (10% w/w) balances debenzylation rate and catalyst cost .
-
Side reactions : Over-hydrogenation of the phenyl ring is mitigated by controlling H₂ pressure (<50 psi) .
Stereochemical Considerations
-
Racemic intermediates necessitate chiral resolution (e.g., enzymatic or chromatographic) to isolate the bioactive R-enantiomer .
Industrial-Scale Adaptations
Continuous Flow Synthesis
-
Microreactors reduce reaction times for oxidation (from 4 hours to 30 minutes) .
-
In-line HPLC monitoring ensures real-time quality control.
Green Chemistry Metrics
Properties
IUPAC Name |
2-[3-[di(propan-2-yl)amino]-1-phenylpropyl]-4-(hydroxymethyl)phenol | |
---|---|---|
Source | PubChem | |
URL | https://pubchem.ncbi.nlm.nih.gov | |
Description | Data deposited in or computed by PubChem | |
InChI |
InChI=1S/C22H31NO2/c1-16(2)23(17(3)4)13-12-20(19-8-6-5-7-9-19)21-14-18(15-24)10-11-22(21)25/h5-11,14,16-17,20,24-25H,12-13,15H2,1-4H3 | |
Source | PubChem | |
URL | https://pubchem.ncbi.nlm.nih.gov | |
Description | Data deposited in or computed by PubChem | |
InChI Key |
DUXZAXCGJSBGDW-UHFFFAOYSA-N | |
Source | PubChem | |
URL | https://pubchem.ncbi.nlm.nih.gov | |
Description | Data deposited in or computed by PubChem | |
Canonical SMILES |
CC(C)N(CCC(C1=CC=CC=C1)C2=C(C=CC(=C2)CO)O)C(C)C | |
Source | PubChem | |
URL | https://pubchem.ncbi.nlm.nih.gov | |
Description | Data deposited in or computed by PubChem | |
Molecular Formula |
C22H31NO2 | |
Source | PubChem | |
URL | https://pubchem.ncbi.nlm.nih.gov | |
Description | Data deposited in or computed by PubChem | |
DSSTOX Substance ID |
DTXSID80432981 | |
Record name | 2-{3-[Di(propan-2-yl)amino]-1-phenylpropyl}-4-(hydroxymethyl)phenol | |
Source | EPA DSSTox | |
URL | https://comptox.epa.gov/dashboard/DTXSID80432981 | |
Description | DSSTox provides a high quality public chemistry resource for supporting improved predictive toxicology. | |
Molecular Weight |
341.5 g/mol | |
Source | PubChem | |
URL | https://pubchem.ncbi.nlm.nih.gov | |
Description | Data deposited in or computed by PubChem | |
CAS No. |
200801-70-3 | |
Record name | 2-{3-[Di(propan-2-yl)amino]-1-phenylpropyl}-4-(hydroxymethyl)phenol | |
Source | EPA DSSTox | |
URL | https://comptox.epa.gov/dashboard/DTXSID80432981 | |
Description | DSSTox provides a high quality public chemistry resource for supporting improved predictive toxicology. | |
Synthesis routes and methods I
Procedure details
Synthesis routes and methods II
Procedure details
Synthesis routes and methods III
Procedure details
Retrosynthesis Analysis
AI-Powered Synthesis Planning: Our tool employs the Template_relevance Pistachio, Template_relevance Bkms_metabolic, Template_relevance Pistachio_ringbreaker, Template_relevance Reaxys, Template_relevance Reaxys_biocatalysis model, leveraging a vast database of chemical reactions to predict feasible synthetic routes.
One-Step Synthesis Focus: Specifically designed for one-step synthesis, it provides concise and direct routes for your target compounds, streamlining the synthesis process.
Accurate Predictions: Utilizing the extensive PISTACHIO, BKMS_METABOLIC, PISTACHIO_RINGBREAKER, REAXYS, REAXYS_BIOCATALYSIS database, our tool offers high-accuracy predictions, reflecting the latest in chemical research and data.
Strategy Settings
Precursor scoring | Relevance Heuristic |
---|---|
Min. plausibility | 0.01 |
Model | Template_relevance |
Template Set | Pistachio/Bkms_metabolic/Pistachio_ringbreaker/Reaxys/Reaxys_biocatalysis |
Top-N result to add to graph | 6 |
Feasible Synthetic Routes
Disclaimer and Information on In-Vitro Research Products
Please be aware that all articles and product information presented on BenchChem are intended solely for informational purposes. The products available for purchase on BenchChem are specifically designed for in-vitro studies, which are conducted outside of living organisms. In-vitro studies, derived from the Latin term "in glass," involve experiments performed in controlled laboratory settings using cells or tissues. It is important to note that these products are not categorized as medicines or drugs, and they have not received approval from the FDA for the prevention, treatment, or cure of any medical condition, ailment, or disease. We must emphasize that any form of bodily introduction of these products into humans or animals is strictly prohibited by law. It is essential to adhere to these guidelines to ensure compliance with legal and ethical standards in research and experimentation.