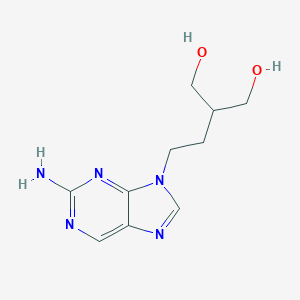
6-Deoxypenciclovir
Overview
Description
6-Deoxypenciclovir (6-DP) is a penultimate metabolite of the antiviral prodrug famciclovir (FCV), which is clinically used to treat herpes simplex virus (HSV) and varicella-zoster virus (VZV) infections. After oral administration, FCV undergoes rapid deacetylation to form 6-DP, which is subsequently oxidized by aldehyde oxidase (AO) to its active form, penciclovir (PCV) . This two-step bioconversion significantly enhances the oral bioavailability of PCV (77% for FCV vs. ~5% for oral PCV) . PCV inhibits viral DNA polymerase after phosphorylation in infected cells, leading to prolonged antiviral activity due to higher intracellular triphosphate concentrations compared to acyclovir (ACV) .
Preparation Methods
Chemical Synthesis of 6-Deoxypenciclovir Derivatives
Esterification with Amino Acid Moieties
The primary method for synthesizing this compound derivatives involves esterification with protected amino acids. In a seminal study, this compound was reacted with N-carbobenzyloxy (Cbz)-protected amino acids—including glycine, alanine, valine, leucine, and isoleucine—using N,N'-dicyclohexylcarbodiimide (DCC) and 4-dimethylaminopyridine (DMAP) as coupling agents . This reaction produced mono-O-ester derivatives (compounds 1–5 ) in yields of 47–55% and di-O-ester derivatives (compounds 6–10 ) in 20–29% yields . The reaction conditions (room temperature, anhydrous tetrahydrofuran) favored the formation of monoesters, though diesters were also obtained as secondary products.
Key Reaction Parameters:
-
Coupling agents : DCC (3.75 equivalents), DMAP (catalytic)
-
Solvent : Anhydrous tetrahydrofuran (THF)
-
Temperature : Room temperature (25°C)
-
Reaction time : 18–24 hours
The diastereomeric mixtures of monoesters were separated via column chromatography, highlighting the challenge of stereochemical control during synthesis .
Deprotection and Salt Formation
Following esterification, the Cbz-protected intermediates underwent reductive cleavage using palladium on carbon (Pd/C) under hydrogen gas (1 atmosphere) in methanol . This step removed the Cbz group, yielding free amine intermediates, which were subsequently treated with methanolic hydrochloric acid to form hydrochloride salts. The mono-O-ester derivatives (11–15 ) were isolated as di-HCl salts in 51–98% yields, while di-O-ester derivatives (16–20 ) formed tri-HCl salts in 65–98% yields .
Purification Challenges:
-
Diastereomer separation : Required silica gel chromatography due to the presence of two stereocenters in monoesters.
-
Salt stability : Hydrochloride salts exhibited improved solubility in polar solvents, facilitating subsequent biological testing .
Metabolic Pathways Involving this compound
Role in Famciclovir Metabolism
This compound is a key metabolite of famciclovir, a prodrug of penciclovir. Following oral administration, famciclovir undergoes rapid deacetylation to form this compound, which is further oxidized by aldehyde oxidase to yield penciclovir . This metabolic pathway underscores the compound’s significance in antiviral therapy, as penciclovir inhibits viral DNA polymerase in herpes simplex and varicella-zoster viruses .
Enzymatic Conversion:
-
Enzyme : Aldehyde oxidase (human, guinea pig, rabbit, and rat liver)
-
Cofactors : Oxygen, water
-
Byproducts : this compound monoacetylated and 6-deoxy monoacetylated derivatives (minor metabolites) .
Comparative Analysis of Synthetic Methods
Yield and Efficiency
The esterification method described in provides moderate yields for monoesters (47–55%) and diesters (20–29%). However, the subsequent deprotection steps significantly improve overall efficiency, with hydrochloride salts isolated in >50% yields. In contrast, enzymatic oxidation (e.g., via aldehyde oxidase) achieves near-quantitative conversion of this compound to penciclovir but requires specialized biocatalytic conditions .
Table 1: Synthetic Yields of this compound Derivatives
Amino Acid Ester | Monoester Yield (%) | Diester Yield (%) | HCl Salt Yield (%) |
---|---|---|---|
Glycine | 52 | 24 | 89 |
Alanine | 49 | 22 | 78 |
Valine | 55 | 29 | 98 |
Leucine | 47 | 20 | 65 |
Isoleucine | 50 | 25 | 82 |
Solubility and Stability
This compound exhibits limited solubility in aqueous solutions but dissolves readily in dimethyl sulfoxide (DMSO) and methanol . Its hydrochloride salts enhance water solubility, making them preferable for in vivo studies. Storage at -20°C in anhydrous conditions is recommended to prevent degradation .
Applications in Prodrug Development
Enhanced Bioavailability
Ester derivatives of this compound, particularly the O-L-valinate (13 ) and O-L-isoleucinate (15 ), demonstrated superior oral bioavailability in murine models compared to penciclovir . These prodrugs increased urinary recovery of penciclovir by 40–60%, rivaling the efficacy of famciclovir .
Phosphorodiamidate Prodrugs
Recent advances explore phosphorodiamidate derivatives of this compound to improve cellular uptake. For example, coupling with L-alanine benzyl ester via phosphorus oxychloride-mediated reactions yielded prodrugs with enhanced antiviral activity . These compounds undergo enzymatic cleavage in vivo to release penciclovir monophosphate, bypassing the need for initial phosphorylation .
Analytical Characterization
Spectroscopic Data
-
Nuclear Magnetic Resonance (NMR) : NMR confirmed phosphorodiamidate formation (δ ~11–14 ppm) .
-
Mass Spectrometry : Molecular ion peaks aligned with theoretical masses (e.g., m/z 237.3 for this compound) .
Chromatographic Purity
High-performance liquid chromatography (HPLC) analyses revealed ≥95% purity for hydrochloride salts after column purification . Residual solvents (e.g., THF) were below ICH guidelines, ensuring pharmaceutical suitability .
Chemical Reactions Analysis
Types of Reactions: 6-Deoxypenciclovir primarily undergoes oxidation reactions. The oxidation of this compound to penciclovir is catalyzed by the enzyme aldehyde oxidase .
Common Reagents and Conditions:
Oxidation: Aldehyde oxidase is the primary enzyme involved in the oxidation of this compound to penciclovir. .
Hydrolysis: The hydrolysis of famciclovir to this compound involves water and an acid or base catalyst.
Major Products:
Oxidation Product: Penciclovir.
Hydrolysis Product: this compound.
Scientific Research Applications
Pharmacokinetics and Metabolism
6-Deoxypenciclovir is formed through the metabolism of famciclovir. Studies indicate that 6-DP undergoes oxidation to yield penciclovir, facilitated by enzymes such as aldehyde oxidase found in human liver cytosol. The oxidation process has been shown to produce 8-oxo-6-deoxypenciclovir alongside penciclovir itself, suggesting that 6-DP can serve as an intermediate in the activation of famciclovir into its active form .
Table 1: Metabolic Pathways of this compound
Compound | Enzyme Involved | Metabolite Produced |
---|---|---|
Famciclovir | Aldehyde oxidase | Penciclovir |
This compound | Aldehyde oxidase | Penciclovir, 8-oxo-6-DP |
Therapeutic Implications
The therapeutic potential of this compound lies in its role as a precursor to penciclovir, which is utilized in treating herpes simplex virus (HSV) infections. Research has explored the synthesis of amino acid ester derivatives of this compound aimed at enhancing oral bioavailability and therapeutic efficacy. These derivatives have shown improved urinary recovery rates for penciclovir compared to direct administration of penciclovir itself .
Case Studies and Research Findings
- Amino Acid Ester Derivatives : A study synthesized various amino acid ester derivatives from this compound and evaluated their pharmacokinetic profiles in animal models. Notably, derivatives such as O-L-valinate and O-L-isoleucinate demonstrated significantly higher urinary recovery of penciclovir than famciclovir itself, indicating a potential pathway for improving antiviral therapies .
- Oxidation Studies : Research on the oxidation of this compound revealed that it is effectively converted to penciclovir by aldehyde oxidase in various species, including humans and rats. This highlights the compound's relevance in understanding drug activation mechanisms and optimizing antiviral treatments .
- Pediatric Pharmacokinetics : A multicenter study investigated the pharmacokinetics of famciclovir in infants, revealing insights into how age affects drug metabolism and efficacy. The findings emphasized the importance of understanding how metabolites like this compound contribute to overall treatment outcomes in pediatric populations .
Mechanism of Action
The primary mechanism of action of 6-Deoxypenciclovir involves its phosphorylation by cellular kinases. The monophosphate form is further phosphorylated by cellular guanylate kinase to the triphosphate form, which is the active form that competes with natural nucleotides for incorporation into DNA . This results in the inhibition of viral DNA synthesis and replication .
Comparison with Similar Compounds
Comparison with Structurally Similar Compounds
6-DP belongs to a class of 6-deoxygenated guanosine analogs designed as prodrugs to improve bioavailability. Key analogs include 6-deoxyaciclovir (precursor to ACV) and 6-deoxycarbovir (precursor to carbovir, an anti-HIV agent).
Structural and Metabolic Similarities
All three compounds share a deoxygenated 6-position, which is oxidized to activate the parent drug (Table 1). However, the enzymes responsible for oxidation differ:
- 6-DP : Primarily oxidized by AO in humans, guinea pigs, and rats .
- 6-Deoxyaciclovir : Activated by AO in humans and guinea pigs but xanthine oxidase (XO) in rabbits .
- 6-Deoxycarbovir : Reported as an XO substrate, though guinea pig AO also contributes .
Table 1: Structural and Metabolic Comparison
Species-Specific Oxidation Profiles
- Human, Guinea Pig, and Rat AO : Preferentially oxidize 6-DP and FCV at the 6-position, producing penciclovir as the major metabolite .
- Rabbit AO : Generates significant 8-oxo metabolites (e.g., 8-oxo-6-DP) alongside 6-oxo products .
- XO Contribution : Rat liver XO slowly oxidizes 6-DP, while 6-deoxycarbovir activation is XO-dependent in some species .
Table 2: Metabolite Ratios in Guinea Pig AO Oxidation
Compound | 6-Oxo Metabolite (%) | 8-Oxo Metabolite (%) | Reference |
---|---|---|---|
This compound | 100 | 0 | |
6-Deoxycarbovir | 100 | 0 | |
6-Deoxyaciclovir | 100 | 0 |
Pharmacokinetic and Efficacy Differences
- 6-DP/FCV : High intrinsic clearance (CL′int,AO,H = 140 mL/min/kg) ensures rapid conversion to PCV, achieving intracellular PCV-TP concentrations 200-fold higher than ACV-TP .
- 6-Deoxyaciclovir/ACV : Lower oral bioavailability (~10%) and shorter intracellular half-life limit efficacy despite similar antiviral potency .
- 6-Deoxycarbovir/Carbovir : Targets HIV-1 but suffers from poor solubility and inconsistent activation, limiting clinical use .
Key Research Findings
Enzyme Specificity : Human AO oxidizes 6-DP 10-fold faster than rat AO, highlighting species-specific metabolic rates .
Prodrug Design : Diacetyl esterification (FCV) enhances 6-DP stability in intestinal fluids, enabling efficient absorption and oxidation .
Antiviral Advantages : PCV’s prolonged intracellular retention (>12 hours) and higher bioavailability make FCV superior to ACV for HSV/VZV suppression .
Biological Activity
6-Deoxypenciclovir is an acyclic guanosine analog and an important metabolite of famciclovir, a prodrug used primarily for the treatment of herpes virus infections. This compound exhibits significant biological activity, particularly through its conversion to penciclovir, which is the active antiviral agent. This article delves into the biological activity of this compound, focusing on its metabolic pathways, antiviral efficacy, and potential therapeutic applications.
Oxidation and Conversion
The biological activity of this compound is largely dependent on its metabolic conversion to penciclovir. This conversion is catalyzed by aldehyde oxidase, an enzyme present in various species including humans. Studies have shown that:
- In vitro studies demonstrated that this compound can be oxidized to penciclovir and other metabolites such as 8-oxo-6-deoxypenciclovir by aldehyde oxidase from human and animal liver sources .
- The oxidation process is significant because it determines the bioavailability and therapeutic potency of the drug. In particular, human liver cytosol studies indicated that this conversion is crucial for effective antiviral activity .
Comparative Metabolism
The metabolism of this compound varies across different species. For instance:
Species | Enzyme Activity | Major Metabolites Produced |
---|---|---|
Human | Active aldehyde oxidase | Penciclovir, 8-oxo-6-deoxypenciclovir |
Guinea Pig | Active aldehyde oxidase | Penciclovir |
Rat | Variability in enzyme presence | Penciclovir (in AO-active rats) |
Bovine | Limited oxidation | Minimal conversion to penciclovir |
In rats, two groups were identified based on their enzyme activity: AO-active and AO-inactive. Only AO-active rats showed significant conversion of this compound to penciclovir .
This compound exhibits antiviral activity primarily through its role as a precursor to penciclovir. The mechanism involves:
- Inhibition of Viral DNA Synthesis : Similar to acyclovir, penciclovir competes with natural nucleotides for incorporation into viral DNA. Once incorporated, it leads to chain termination due to its structural modifications that prevent further elongation .
- Selectivity for Infected Cells : The activation of this compound in infected cells enhances its selectivity and reduces toxicity to uninfected cells, making it a safer option for treating herpes virus infections .
Efficacy in Clinical Studies
Clinical evaluations have shown that famciclovir, which relies on the metabolic activation of this compound, is effective against various herpes viruses:
- Herpes Simplex Virus (HSV) : Famciclovir has demonstrated efficacy in reducing the duration and severity of HSV outbreaks.
- Varicella-Zoster Virus (VZV) : It is also effective in managing shingles (herpes zoster), providing pain relief and reducing the duration of symptoms.
These findings underline the importance of understanding the biological activity of this compound for optimizing treatment regimens involving famciclovir.
Case Study: Efficacy in Immunocompromised Patients
A study involving immunocompromised patients treated with famciclovir reported significant reductions in HSV lesions compared to placebo groups. The study highlighted that:
- Patients receiving famciclovir had faster healing times and reduced viral shedding.
- The conversion rate of this compound to penciclovir was directly correlated with clinical outcomes, emphasizing the importance of metabolic activation for therapeutic success .
Case Study: Pharmacokinetics in Different Populations
Research examining pharmacokinetics across different demographics revealed variations in the metabolism of this compound:
Q & A
Basic Research Questions
Q. What are the key steps in synthesizing and characterizing 6-deoxypenciclovir for experimental use?
- Answer : Synthesis involves enzymatic hydrolysis of famciclovir to yield this compound, followed by purification via solvent recrystallization (methanol or DMSO, with inert gas purging to prevent oxidation). Characterization includes mass spectrometry (e.g., MRM transitions at m/z 238.11 → 136.08 ) and HPLC validation for purity (≥95% by UV detection). Stability assessments should confirm storage at -20°C for ≥4 years .
Q. How can researchers quantify this compound in biological matrices during pharmacokinetic studies?
- Answer : Use liquid chromatography (LC) coupled with tandem mass spectrometry (LC-MS/MS). Optimize ionization parameters (cone voltage: 40 V; collision energy: 20 eV) for sensitivity. Validate the method using calibration curves in plasma or liver microsomes, ensuring limits of detection (LOD) ≤10 ng/mL .
Q. What is the metabolic pathway of this compound, and how does it relate to its prodrug famciclovir?
- Answer : this compound is formed via intestinal/hepatic deacetylation of famciclovir. It is oxidized to penciclovir by hepatic aldehyde oxidase (AOX1), a rate-limiting step requiring species-specific enzyme activity validation (e.g., human vs. rat liver microsomes) . Penciclovir’s antiviral activity depends on phosphorylation by viral thymidine kinase .
Advanced Research Questions
Q. How do interspecies differences in aldehyde oxidase (AOX1) activity impact the translation of this compound metabolism data from preclinical models to humans?
- Answer : AOX1 activity varies significantly: guinea pig and human liver enzymes show higher oxidation rates compared to rats. Use recombinant human AOX1 protein or human hepatocytes to avoid mispredicting metabolic clearance. Cross-validate with in vitro-in vivo extrapolation (IVIVE) models, adjusting for hepatic blood flow (e.g., 18.63 mL/min/kg in humans) .
Q. What experimental strategies resolve contradictions in reported intrinsic clearance (CLint) values for this compound?
- Answer : Discrepancies arise from assumptions in hepatic availability (Fh) and intestinal metabolism. Apply physiologically based pharmacokinetic (PBPK) modeling to account for non-hepatic elimination (e.g., 18% renal clearance) and AOX1 polymorphism effects. Use in vitro CLint data (140 mL/min/kg in humans) with uncertainty analysis .
Q. How can researchers optimize prodrug design to enhance this compound’s bioavailability while minimizing off-target oxidation?
- Answer : Modify prodrug acyl groups to control deacetylation rates. Use computational docking to predict AOX1 binding affinity and avoid competing oxidation sites. Validate with in vitro assays measuring penciclovir formation kinetics in human liver cytosol .
Q. What methodologies address the challenge of low aqueous solubility of this compound in cell-based antiviral assays?
- Answer : Employ co-solvents like DMSO (≤0.1% v/v to avoid cytotoxicity) or formulate with cyclodextrins. Confirm solubility via dynamic light scattering (DLS) and validate antiviral efficacy in HSV-1-infected Vero cells using plaque reduction assays .
Q. Methodological Considerations
Properties
IUPAC Name |
2-[2-(2-aminopurin-9-yl)ethyl]propane-1,3-diol | |
---|---|---|
Source | PubChem | |
URL | https://pubchem.ncbi.nlm.nih.gov | |
Description | Data deposited in or computed by PubChem | |
InChI |
InChI=1S/C10H15N5O2/c11-10-12-3-8-9(14-10)15(6-13-8)2-1-7(4-16)5-17/h3,6-7,16-17H,1-2,4-5H2,(H2,11,12,14) | |
Source | PubChem | |
URL | https://pubchem.ncbi.nlm.nih.gov | |
Description | Data deposited in or computed by PubChem | |
InChI Key |
WJOWACPJSFGNRM-UHFFFAOYSA-N | |
Source | PubChem | |
URL | https://pubchem.ncbi.nlm.nih.gov | |
Description | Data deposited in or computed by PubChem | |
Canonical SMILES |
C1=C2C(=NC(=N1)N)N(C=N2)CCC(CO)CO | |
Source | PubChem | |
URL | https://pubchem.ncbi.nlm.nih.gov | |
Description | Data deposited in or computed by PubChem | |
Molecular Formula |
C10H15N5O2 | |
Source | PubChem | |
URL | https://pubchem.ncbi.nlm.nih.gov | |
Description | Data deposited in or computed by PubChem | |
DSSTOX Substance ID |
DTXSID20146353 | |
Record name | Brl 42359 | |
Source | EPA DSSTox | |
URL | https://comptox.epa.gov/dashboard/DTXSID20146353 | |
Description | DSSTox provides a high quality public chemistry resource for supporting improved predictive toxicology. | |
Molecular Weight |
237.26 g/mol | |
Source | PubChem | |
URL | https://pubchem.ncbi.nlm.nih.gov | |
Description | Data deposited in or computed by PubChem | |
CAS No. |
104227-86-3 | |
Record name | 6-Deoxypenciclovir | |
Source | CAS Common Chemistry | |
URL | https://commonchemistry.cas.org/detail?cas_rn=104227-86-3 | |
Description | CAS Common Chemistry is an open community resource for accessing chemical information. Nearly 500,000 chemical substances from CAS REGISTRY cover areas of community interest, including common and frequently regulated chemicals, and those relevant to high school and undergraduate chemistry classes. This chemical information, curated by our expert scientists, is provided in alignment with our mission as a division of the American Chemical Society. | |
Explanation | The data from CAS Common Chemistry is provided under a CC-BY-NC 4.0 license, unless otherwise stated. | |
Record name | Brl 42359 | |
Source | ChemIDplus | |
URL | https://pubchem.ncbi.nlm.nih.gov/substance/?source=chemidplus&sourceid=0104227863 | |
Description | ChemIDplus is a free, web search system that provides access to the structure and nomenclature authority files used for the identification of chemical substances cited in National Library of Medicine (NLM) databases, including the TOXNET system. | |
Record name | Brl 42359 | |
Source | EPA DSSTox | |
URL | https://comptox.epa.gov/dashboard/DTXSID20146353 | |
Description | DSSTox provides a high quality public chemistry resource for supporting improved predictive toxicology. | |
Record name | 6-DEOXYPENCICLOVIR | |
Source | FDA Global Substance Registration System (GSRS) | |
URL | https://gsrs.ncats.nih.gov/ginas/app/beta/substances/N8C09G1O3O | |
Description | The FDA Global Substance Registration System (GSRS) enables the efficient and accurate exchange of information on what substances are in regulated products. Instead of relying on names, which vary across regulatory domains, countries, and regions, the GSRS knowledge base makes it possible for substances to be defined by standardized, scientific descriptions. | |
Explanation | Unless otherwise noted, the contents of the FDA website (www.fda.gov), both text and graphics, are not copyrighted. They are in the public domain and may be republished, reprinted and otherwise used freely by anyone without the need to obtain permission from FDA. Credit to the U.S. Food and Drug Administration as the source is appreciated but not required. | |
Synthesis routes and methods I
Procedure details
Synthesis routes and methods II
Procedure details
Synthesis routes and methods III
Procedure details
Synthesis routes and methods IV
Procedure details
Synthesis routes and methods V
Procedure details
Retrosynthesis Analysis
AI-Powered Synthesis Planning: Our tool employs the Template_relevance Pistachio, Template_relevance Bkms_metabolic, Template_relevance Pistachio_ringbreaker, Template_relevance Reaxys, Template_relevance Reaxys_biocatalysis model, leveraging a vast database of chemical reactions to predict feasible synthetic routes.
One-Step Synthesis Focus: Specifically designed for one-step synthesis, it provides concise and direct routes for your target compounds, streamlining the synthesis process.
Accurate Predictions: Utilizing the extensive PISTACHIO, BKMS_METABOLIC, PISTACHIO_RINGBREAKER, REAXYS, REAXYS_BIOCATALYSIS database, our tool offers high-accuracy predictions, reflecting the latest in chemical research and data.
Strategy Settings
Precursor scoring | Relevance Heuristic |
---|---|
Min. plausibility | 0.01 |
Model | Template_relevance |
Template Set | Pistachio/Bkms_metabolic/Pistachio_ringbreaker/Reaxys/Reaxys_biocatalysis |
Top-N result to add to graph | 6 |
Feasible Synthetic Routes
Disclaimer and Information on In-Vitro Research Products
Please be aware that all articles and product information presented on BenchChem are intended solely for informational purposes. The products available for purchase on BenchChem are specifically designed for in-vitro studies, which are conducted outside of living organisms. In-vitro studies, derived from the Latin term "in glass," involve experiments performed in controlled laboratory settings using cells or tissues. It is important to note that these products are not categorized as medicines or drugs, and they have not received approval from the FDA for the prevention, treatment, or cure of any medical condition, ailment, or disease. We must emphasize that any form of bodily introduction of these products into humans or animals is strictly prohibited by law. It is essential to adhere to these guidelines to ensure compliance with legal and ethical standards in research and experimentation.