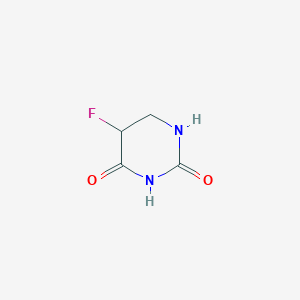
5-Fluorodihydrouracil
Overview
Description
5,6-Dihydro-5-Fluorouracil is an active metabolite of the thymidylate synthase inhibitor prodrug 5-fluorouracil. It is formed from 5-fluorouracil by the action of the enzyme dihydropyrimidine dehydrogenase. This compound is known for its cytotoxic properties, particularly against certain types of cancer cells .
Mechanism of Action
Target of Action
5-Fluorodihydrouracil (5-FU) primarily targets the enzyme thymidylate synthase (TS) . This enzyme plays a crucial role in DNA synthesis by converting deoxyuridylic acid to thymidylic acid . Another key target is dihydropyrimidine dehydrogenase (DPD) , which is responsible for converting approximately 85% of 5-FU into inactive this compound .
Mode of Action
5-FU is a pyrimidine analog that inhibits RNA and DNA synthesis through several mechanisms . The main mechanism of action is thought to be the binding of the deoxyribonucleotide of the drug (FdUMP) and the folate cofactor, N5–10-methylenetetrahydrofolate, to TS to form a covalently bound ternary complex . This binding inhibits the function of TS, thereby disrupting DNA synthesis .
Biochemical Pathways
5-FU affects several biochemical pathways. It is converted intracellularly to several active metabolites: fluorodeoxyuridine monophosphate (FdUMP), fluorodeoxyuridine triphosphate (FdUTP), and fluorouridine triphosphate (FUTP). These active metabolites disrupt RNA synthesis and the action of TS . The complexity of the anabolic and catabolic pathways followed by 5-FU and their variability among tissue types, between normal and tumor tissues, and across individuals underlies the variety of outcomes observed following treatment with 5-FU .
Pharmacokinetics
The pharmacokinetics of 5-FU is characterized by marked interpatient variability. Physiologically based pharmacokinetic (PBPK) models of capecitabine-metabolites and 5-FU-metabolites have been developed to describe their pharmacokinetics in tumor and plasma of cancer patients with liver impairment . These models suggest that no dosage adaptation of capecitabine or 5-FU is required for cancer patients with hepatic impairment .
Result of Action
5-FU has significant effects at the molecular and cellular levels. It inhibits cell proliferation by inducing cell cycle arrest in the G1 phase and triggers apoptosis . It also induces changes in cellular and nuclear morphology, reduces the number of actin-containing stress fibers, and decreases the number of caveolin-3 positive cells .
Action Environment
Environmental factors can influence the action, efficacy, and stability of 5-FU. For instance, the presence of natural compounds like hesperidin and curcumin can protect against 5-FU-induced nephrotoxicity . Additionally, genetic polymorphisms, such as those in the DPYD gene, can lead to decreased metabolism and/or decreased or absent enzyme activity, resulting in increased 5-FU side effects .
Biochemical Analysis
Biochemical Properties
5-Fluorodihydrouracil interacts with several enzymes and proteins within the cell. It is formed from 5-Fluorouracil by the enzyme dihydropyrimidine dehydrogenase (DPD) . The conversion of 5-Fluorouracil to this compound is an important step in the metabolism of the drug, and variations in DPD activity can significantly affect the drug’s effectiveness and toxicity .
Cellular Effects
This compound has a profound impact on various types of cells and cellular processes. It affects cell proliferation, cell cycle, survival, and induces apoptosis . It also influences cell function by modulating cancer-related pathways, cell apoptosis, autophagy, epithelial–mesenchymal transition, and other aspects of cell behavior .
Molecular Mechanism
The molecular mechanism of this compound involves its incorporation into RNA and DNA, inhibiting their normal function . It binds to thymidylate synthase (TS), resulting in inhibition of DNA synthesis and induction of programmed cell death .
Temporal Effects in Laboratory Settings
In laboratory settings, the effects of this compound change over time. It has been observed that the drug’s oral absorption is incomplete with a short biological half-life . This leads to a frequent administration requirement and severe side effects .
Dosage Effects in Animal Models
In animal models, the effects of this compound vary with different dosages. A study showed that this compound was administered at doses of 15 mg/kg for 4 consecutive days, then reduced to 6 mg/kg for 4 alternate days . The study found that men had a higher elimination of the drug compared to women .
Metabolic Pathways
This compound is involved in several metabolic pathways. It is metabolized initially by conversion to this compound by the enzyme dihydropyrimidine dehydrogenase (DPD) . This occurs in all tissues, but its activity is most intense in the liver .
Transport and Distribution
This compound is transported and distributed within cells and tissues. It rapidly enters the cell using the exact facilitated transport mechanism as uracil . The drug’s distribution within the body can be affected by patient-specific factors, such as gender .
Subcellular Localization
It is known that the drug’s active forms, including 5-fluorodeoxyuridylate (5-FdUMP), 5-fluorodeoxyuridine triphosphate (5-FdUTP), or fluorouridine triphosphate (5-FUTP), play crucial roles in its mechanism of action .
Preparation Methods
Synthetic Routes and Reaction Conditions: 5,6-Dihydro-5-Fluorouracil can be synthesized by the hydrogenation of 5-fluorouracil. In one method, 5-fluorouracil is dissolved in methanol and subjected to hydrogenation in the presence of palladium on carbon as a catalyst. The reaction is carried out under a hydrogen atmosphere at room temperature for 36 hours .
Industrial Production Methods: The industrial production of 5,6-dihydro-5-fluorouracil typically involves the enzymatic reduction of 5-fluorouracil using dihydropyrimidine dehydrogenase. This method is preferred due to its higher yield and specificity .
Chemical Reactions Analysis
Types of Reactions: 5,6-Dihydro-5-Fluorouracil primarily undergoes reduction reactions. It is formed by the reduction of 5-fluorouracil and can further participate in various biochemical reactions within the body.
Common Reagents and Conditions:
Hydrogenation: Palladium on carbon (Pd/C) as a catalyst, methanol as a solvent, and hydrogen gas.
Enzymatic Reduction: Dihydropyrimidine dehydrogenase enzyme.
Major Products: The major product of the reduction of 5-fluorouracil is 5,6-dihydro-5-fluorouracil itself. Further metabolism can lead to the formation of other metabolites, such as α-fluoro-β-alanine .
Scientific Research Applications
Clinical Applications in Cancer Treatment
5-Fluorodihydrouracil plays a crucial role in enhancing the efficacy of 5-FU-based chemotherapy regimens. The compound's mechanism involves the inhibition of thymidylate synthase, leading to disrupted DNA synthesis in rapidly dividing cancer cells.
Therapeutic Drug Monitoring (TDM)
TDM of 5-FU and its metabolite FUH2 is essential for optimizing treatment outcomes in patients with colorectal cancer and head and neck cancers. Studies indicate that monitoring plasma levels of 5-FU can significantly reduce toxicity and improve therapeutic efficacy.
- Case Study: Head and Neck Cancer
- Case Study: Metastatic Colorectal Cancer
Combination Chemotherapy Regimens
FUH2 is often utilized in combination with other agents such as cisplatin to enhance the overall effectiveness of chemotherapy. The integration of FUH2 into treatment protocols has shown promising results in improving survival rates and minimizing side effects.
- Example: mFOLFOX6 Protocol
Pharmacokinetics and Biomarkers
Understanding the pharmacokinetics of FUH2 is vital for predicting patient responses to treatment. Research indicates that the ratio of dihydrouracil to uracil (UH2/Ura) may serve as a predictive biomarker for altered pharmacokinetics following repeated administration of 5-FU.
Predictive Biomarkers
A study highlighted the relationship between the UH2/Ura ratio and hepatic dihydropyrimidine dehydrogenase (DPD) activity, suggesting that this biomarker could help tailor treatment regimens based on individual metabolic responses .
Pharmacokinetic Studies
Pharmacokinetic studies have shown that many patients receiving 5-FU do not achieve optimal plasma concentrations, with only 20% to 30% treated within the recommended AUC range. This underscores the importance of TDM in clinical practice .
Toxicological Considerations
While FUH2 is beneficial in cancer therapy, it also presents potential toxicological risks. The compound can cause toxicity to bone marrow and gastrointestinal tracts, necessitating careful monitoring during treatment.
Toxicity Profiles
- Bone Marrow Toxicity : Patients may experience neutropenia and thrombocytopenia as side effects, particularly when dosing is not appropriately managed.
- Gastrointestinal Toxicity : Symptoms can include mucositis and diarrhea, which are common adverse effects associated with high doses or improper dosing regimens .
Management Strategies
To mitigate these risks, clinicians employ strategies such as dose adjustments based on individual patient responses and regular monitoring of blood counts during therapy.
Comparison with Similar Compounds
5-Fluorouracil: The parent compound from which 5,6-dihydro-5-fluorouracil is derived. It is widely used in chemotherapy for various cancers.
Tegafur: A prodrug of 5-fluorouracil that is used in combination with other drugs for cancer treatment.
Capecitabine: Another prodrug of 5-fluorouracil that is used in the treatment of colorectal and breast cancers.
Uniqueness: 5,6-Dihydro-5-Fluorouracil is unique in its specific formation through the reduction of 5-fluorouracil and its role as an active metabolite. Its cytotoxic properties and mechanism of action make it a valuable compound in cancer research and treatment .
Biological Activity
5-Fluorodihydrouracil (FDHU) is a significant metabolite of 5-fluorouracil (5-FU), a widely used chemotherapeutic agent. Understanding the biological activity of FDHU is crucial for optimizing cancer treatments, particularly in patients undergoing therapy with 5-FU. This article explores the pharmacological properties, mechanisms of action, and clinical implications of FDHU, supported by research findings and case studies.
FDHU is formed through the reduction of 5-FU, primarily via the enzyme dihydropyrimidine dehydrogenase (DPD). This conversion is significant as it affects the pharmacokinetics and therapeutic efficacy of 5-FU. The metabolic pathway is illustrated below:
The presence of DPD influences the levels of both 5-FU and FDHU in the body, impacting their respective biological activities. Inhibition or deficiency in DPD can lead to increased toxicity from 5-FU due to higher systemic concentrations.
FDHU exhibits its biological activity primarily through its role in modulating the effects of 5-FU. It has been shown to:
- Inhibit Thymidylate Synthase : Similar to 5-FU, FDHU interferes with DNA synthesis by inhibiting thymidylate synthase, an essential enzyme for DNA replication.
- Induce Apoptosis : FDHU can trigger programmed cell death in cancer cells, enhancing the cytotoxic effects initiated by 5-FU.
- Alter RNA Function : By misincorporating into RNA, FDHU disrupts normal cellular processes, contributing to its antitumor activity.
Toxicity Profile
While FDHU has therapeutic benefits, it also poses risks. Toxicity associated with FDHU includes:
- Bone Marrow Suppression : Increased levels of FDHU can lead to myelosuppression, a common side effect observed in patients receiving 5-FU.
- Gastrointestinal Toxicity : Patients may experience severe gastrointestinal reactions due to elevated FDHU levels.
Clinical Studies and Case Reports
A range of studies has investigated the clinical implications of FDHU in cancer therapy:
- Pharmacokinetic Studies : Research indicates that variations in DPD activity significantly affect the pharmacokinetics of both 5-FU and FDHU. For instance, patients with DPD deficiency exhibited severe toxicity when treated with standard doses of 5-FU due to accumulation of its metabolites, including FDHU .
- Case Studies on Toxicity : In a notable case report, a patient with asymptomatic DPD deficiency developed life-threatening toxicity after administration of 5-FU. The study highlighted the importance of screening for DPD activity prior to treatment .
- Therapeutic Monitoring : Recent advancements in assay techniques allow for better monitoring of FDHU levels in patients' blood samples, facilitating personalized treatment approaches that could mitigate toxicity while enhancing efficacy .
Research Findings
A summary table below illustrates key findings from various studies regarding the biological activity and clinical implications of FDHU:
Properties
IUPAC Name |
5-fluoro-1,3-diazinane-2,4-dione | |
---|---|---|
Source | PubChem | |
URL | https://pubchem.ncbi.nlm.nih.gov | |
Description | Data deposited in or computed by PubChem | |
InChI |
InChI=1S/C4H5FN2O2/c5-2-1-6-4(9)7-3(2)8/h2H,1H2,(H2,6,7,8,9) | |
Source | PubChem | |
URL | https://pubchem.ncbi.nlm.nih.gov | |
Description | Data deposited in or computed by PubChem | |
InChI Key |
RAIRJKWTBBDDAR-UHFFFAOYSA-N | |
Source | PubChem | |
URL | https://pubchem.ncbi.nlm.nih.gov | |
Description | Data deposited in or computed by PubChem | |
Canonical SMILES |
C1C(C(=O)NC(=O)N1)F | |
Source | PubChem | |
URL | https://pubchem.ncbi.nlm.nih.gov | |
Description | Data deposited in or computed by PubChem | |
Molecular Formula |
C4H5FN2O2 | |
Source | PubChem | |
URL | https://pubchem.ncbi.nlm.nih.gov | |
Description | Data deposited in or computed by PubChem | |
DSSTOX Substance ID |
DTXSID90989678 | |
Record name | 5-Fluoro-5,6-dihydropyrimidine-2,4-diol | |
Source | EPA DSSTox | |
URL | https://comptox.epa.gov/dashboard/DTXSID90989678 | |
Description | DSSTox provides a high quality public chemistry resource for supporting improved predictive toxicology. | |
Molecular Weight |
132.09 g/mol | |
Source | PubChem | |
URL | https://pubchem.ncbi.nlm.nih.gov | |
Description | Data deposited in or computed by PubChem | |
CAS No. |
696-06-0 | |
Record name | 5,6-Dihydro-5-fluorouracil | |
Source | CAS Common Chemistry | |
URL | https://commonchemistry.cas.org/detail?cas_rn=696-06-0 | |
Description | CAS Common Chemistry is an open community resource for accessing chemical information. Nearly 500,000 chemical substances from CAS REGISTRY cover areas of community interest, including common and frequently regulated chemicals, and those relevant to high school and undergraduate chemistry classes. This chemical information, curated by our expert scientists, is provided in alignment with our mission as a division of the American Chemical Society. | |
Explanation | The data from CAS Common Chemistry is provided under a CC-BY-NC 4.0 license, unless otherwise stated. | |
Record name | 5-Fluorodihydrouracil | |
Source | ChemIDplus | |
URL | https://pubchem.ncbi.nlm.nih.gov/substance/?source=chemidplus&sourceid=0000696060 | |
Description | ChemIDplus is a free, web search system that provides access to the structure and nomenclature authority files used for the identification of chemical substances cited in National Library of Medicine (NLM) databases, including the TOXNET system. | |
Record name | 5-Fluoro-5,6-dihydropyrimidine-2,4-diol | |
Source | EPA DSSTox | |
URL | https://comptox.epa.gov/dashboard/DTXSID90989678 | |
Description | DSSTox provides a high quality public chemistry resource for supporting improved predictive toxicology. | |
Record name | 2,4(1H,3H)-Pyrimidinedione, 5-fluorodihydro | |
Source | European Chemicals Agency (ECHA) | |
URL | https://echa.europa.eu/information-on-chemicals | |
Description | The European Chemicals Agency (ECHA) is an agency of the European Union which is the driving force among regulatory authorities in implementing the EU's groundbreaking chemicals legislation for the benefit of human health and the environment as well as for innovation and competitiveness. | |
Explanation | Use of the information, documents and data from the ECHA website is subject to the terms and conditions of this Legal Notice, and subject to other binding limitations provided for under applicable law, the information, documents and data made available on the ECHA website may be reproduced, distributed and/or used, totally or in part, for non-commercial purposes provided that ECHA is acknowledged as the source: "Source: European Chemicals Agency, http://echa.europa.eu/". Such acknowledgement must be included in each copy of the material. ECHA permits and encourages organisations and individuals to create links to the ECHA website under the following cumulative conditions: Links can only be made to webpages that provide a link to the Legal Notice page. | |
Record name | 5-FLUORO-5,6-DIHYDROURACIL | |
Source | FDA Global Substance Registration System (GSRS) | |
URL | https://gsrs.ncats.nih.gov/ginas/app/beta/substances/CLP4LM9HXK | |
Description | The FDA Global Substance Registration System (GSRS) enables the efficient and accurate exchange of information on what substances are in regulated products. Instead of relying on names, which vary across regulatory domains, countries, and regions, the GSRS knowledge base makes it possible for substances to be defined by standardized, scientific descriptions. | |
Explanation | Unless otherwise noted, the contents of the FDA website (www.fda.gov), both text and graphics, are not copyrighted. They are in the public domain and may be republished, reprinted and otherwise used freely by anyone without the need to obtain permission from FDA. Credit to the U.S. Food and Drug Administration as the source is appreciated but not required. | |
Retrosynthesis Analysis
AI-Powered Synthesis Planning: Our tool employs the Template_relevance Pistachio, Template_relevance Bkms_metabolic, Template_relevance Pistachio_ringbreaker, Template_relevance Reaxys, Template_relevance Reaxys_biocatalysis model, leveraging a vast database of chemical reactions to predict feasible synthetic routes.
One-Step Synthesis Focus: Specifically designed for one-step synthesis, it provides concise and direct routes for your target compounds, streamlining the synthesis process.
Accurate Predictions: Utilizing the extensive PISTACHIO, BKMS_METABOLIC, PISTACHIO_RINGBREAKER, REAXYS, REAXYS_BIOCATALYSIS database, our tool offers high-accuracy predictions, reflecting the latest in chemical research and data.
Strategy Settings
Precursor scoring | Relevance Heuristic |
---|---|
Min. plausibility | 0.01 |
Model | Template_relevance |
Template Set | Pistachio/Bkms_metabolic/Pistachio_ringbreaker/Reaxys/Reaxys_biocatalysis |
Top-N result to add to graph | 6 |
Feasible Synthetic Routes
Q1: How does 5-fluorodihydrouracil fit into the metabolic pathway of capecitabine?
A1: Capecitabine, an orally administered chemotherapy drug, undergoes a series of enzymatic conversions within the body. It is first metabolized to 5′-deoxy-5-fluorocytidine (5′-DFCR) and subsequently to 5′-deoxy-5-fluorouridine (5′-DFUR). 5′-DFUR is then further metabolized into 5-fluorouracil (5-FU), the active metabolite. This compound (5-FUH2) is a major metabolite of 5-FU, formed through the action of the enzyme dihydropyrimidine dehydrogenase (DPD). [, ]
Q2: Why is the simultaneous measurement of capecitabine and its metabolites important?
A2: Understanding the pharmacokinetic profile of capecitabine, including the levels of its metabolites, is crucial for optimizing treatment and predicting potential toxicity. This is particularly important because 5-FU exhibits a narrow therapeutic index. Several studies have employed sensitive analytical methods, such as liquid chromatography-mass spectrometry (LC-MS), to simultaneously quantify capecitabine, 5′-DFCR, 5′-DFUR, 5-FU, and 5-FUH2 in human plasma. [, ] These methods offer high sensitivity and selectivity, enabling researchers to accurately monitor the drug and its metabolites in patients undergoing treatment. [, ]
Q3: What are the analytical challenges in measuring this compound alongside other metabolites?
A3: The accurate and reliable quantification of this compound and other capecitabine metabolites in biological matrices, such as plasma, presents significant analytical challenges. These challenges stem from the low concentrations of these compounds in biological samples, the complexity of the matrix, and the potential for interference from endogenous compounds. To overcome these challenges, researchers have developed sophisticated analytical techniques like LC-MS. [, ] This method involves separating the analytes based on their physicochemical properties using liquid chromatography, followed by their detection and quantification using mass spectrometry.
Q4: How does the metabolism of 5-fluorocytosine relate to this compound?
A4: While both are fluoropyrimidine compounds, 5-fluorocytosine (5-FC) follows a different metabolic pathway than capecitabine. Interestingly, 5-FC can be converted into 5-FU, ultimately leading to the formation of 5-FUH2. [] This metabolic conversion is important to consider, especially when patients are treated with both drugs.
Q5: Are there differences in the metabolism of these compounds across species?
A5: Research indicates that the metabolism of 5-fluorocytosine varies among species. Studies have shown that humans exhibit lower levels of 5-FC metabolism compared to other animals like mice, rats, rabbits, and dogs. [] This interspecies variability in drug metabolism underscores the importance of using appropriate models when studying these compounds.
Disclaimer and Information on In-Vitro Research Products
Please be aware that all articles and product information presented on BenchChem are intended solely for informational purposes. The products available for purchase on BenchChem are specifically designed for in-vitro studies, which are conducted outside of living organisms. In-vitro studies, derived from the Latin term "in glass," involve experiments performed in controlled laboratory settings using cells or tissues. It is important to note that these products are not categorized as medicines or drugs, and they have not received approval from the FDA for the prevention, treatment, or cure of any medical condition, ailment, or disease. We must emphasize that any form of bodily introduction of these products into humans or animals is strictly prohibited by law. It is essential to adhere to these guidelines to ensure compliance with legal and ethical standards in research and experimentation.