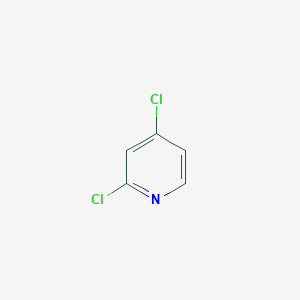
2,4-Dichloropyridine
Overview
Description
2,4-Dichloropyridine (C₅H₃Cl₂N) is a heteroaromatic compound featuring two chlorine atoms at the 2- and 4-positions of the pyridine ring. Its molecular structure confers distinct electronic and steric properties, making it a versatile intermediate in pharmaceutical, agrochemical, and materials chemistry. The compound’s reactivity is governed by the electron-withdrawing effects of chlorine substituents, which activate specific positions for nucleophilic or electrophilic substitution. For example, nucleophilic substitution occurs preferentially at the 4-position due to reduced steric hindrance and favorable resonance stabilization .
Preparation Methods
Synthetic Routes and Reaction Conditions: 2,4-Dichloropyridine can be synthesized through several methods:
Direct Chlorination of Pyridine: Pyridine is chlorinated using chlorine gas in the presence of a catalyst such as iron(III) chloride.
Chlorination of Pyridine-N-Oxides: Pyridine-N-oxides can be chlorinated using reagents like phosphoryl chloride to yield this compound.
Industrial Production Methods: Industrial production of this compound often involves the direct chlorination of pyridine due to its simplicity and cost-effectiveness. The process is optimized to maximize yield and minimize by-products.
Chemical Reactions Analysis
Types of Reactions:
Nucleophilic Substitution: 2,4-Dichloropyridine undergoes nucleophilic substitution reactions where the chlorine atoms are replaced by nucleophiles such as amines, thiols, or alkoxides.
Suzuki Coupling: This compound can participate in Suzuki coupling reactions with boronic acids to form biaryl compounds.
Common Reagents and Conditions:
Nucleophilic Substitution: Common reagents include sodium methoxide, potassium thiolate, and primary amines.
Suzuki Coupling: Palladium catalysts, such as palladium(II) acetate, are used along with bases like potassium carbonate in solvents like ethanol or toluene.
Major Products:
Nucleophilic Substitution: Products include substituted pyridines where the chlorine atoms are replaced by the nucleophile.
Suzuki Coupling: Products are biaryl compounds with various functional groups depending on the boronic acid used.
Scientific Research Applications
Chemical Synthesis
2,4-Dichloropyridine serves as an important building block in the synthesis of various organic compounds. Its chlorinated structure allows for selective reactions, making it a valuable intermediate in many chemical processes.
1.1. Palladium-Catalyzed Reactions
One of the notable applications of this compound is in palladium-catalyzed reactions. For instance, a highly regioselective C-2 amination process was developed using palladium(0) catalysts. This method allows for the coupling of this compound with aminoarenes to produce 4-chloro-6-anilino nicotinonitrile compounds after deprotection . This reaction highlights the compound's utility in synthesizing complex molecules with high regioselectivity.
1.2. Suzuki Coupling Reactions
Another significant application is in Suzuki coupling reactions. This compound can undergo effective one-pot double Suzuki coupling to yield diarylated products. The choice of solvent plays a crucial role in optimizing these reactions, demonstrating the compound's adaptability in synthetic methodologies .
Pharmaceutical Applications
The pharmaceutical industry recognizes this compound for its potential in drug development. Its derivatives have been explored for various biological activities.
2.1. Anticancer Agents
Research has indicated that derivatives of this compound exhibit promising anticancer properties. For example, studies have synthesized compounds that target specific cancer pathways, showcasing the compound's role as a precursor in developing novel anticancer agents .
2.2. Antidepressants and CNS Agents
The compound has also been investigated for its potential as a central nervous system (CNS) agent. Certain derivatives have shown activity as selective antagonists for corticotropin-releasing factor receptors, indicating possible applications in treating anxiety and depression .
Agrochemical Applications
In agrochemistry, this compound is utilized in the formulation of herbicides and pesticides.
3.1. Herbicide Development
The compound's structure allows it to serve as a precursor for herbicides that target specific plant growth mechanisms. Its derivatives have been tested for efficacy against various weed species, contributing to integrated pest management strategies .
Material Science Applications
This compound is also applied in material science, particularly in the development of dyes and pigments.
4.1. Dye Synthesis
The compound contributes to the formulation of vibrant dyes due to its ability to participate in electrophilic substitution reactions. This application is crucial for industries focused on textile and polymer manufacturing .
Case Studies and Research Findings
Mechanism of Action
The mechanism of action of 2,4-dichloropyridine depends on its specific application. In medicinal chemistry, it often acts as a precursor to bioactive molecules that interact with specific molecular targets such as enzymes or receptors. The chlorine atoms in the pyridine ring can enhance the compound’s binding affinity and selectivity towards these targets .
Comparison with Similar Compounds
Structural and Electronic Differences
2,6-Dichloropyridine
- Structure : Chlorines at 2- and 6-positions create symmetrical steric hindrance.
- Reactivity : Exhibits lower reactivity in cross-coupling reactions compared to 2,4-dichloropyridine. Ligand-free Suzuki-Miyaura coupling of this compound achieves >99:1 C4-selectivity, while 2,6-dichloropyridine derivatives are less explored due to steric constraints .
- Applications : Used in synthesizing pyrazolopyridines (e.g., via lithiation and aldehyde trapping) but lacks the regioselectivity seen in this compound .
3,5-Dichloropyridine
- Structure : Chlorines at meta-positions relative to nitrogen.
- Reactivity : Demonstrates unique selectivity in medicinal chemistry. For instance, 3,5-dichloropyridine derivatives act as potent P2X7 receptor antagonists (IC₅₀ = 4.9 nM) due to optimized hydrophobic interactions, unlike this compound’s applications in cross-coupling .
- Electronic Effects : The meta-chlorines reduce ring electron density more uniformly, limiting electrophilic substitution compared to this compound.
2-Chloropyridine
- Structure : Single chlorine at the 2-position.
- Reactivity : 70-fold more reactive with thiols than 4-chloropyridine due to proximity to the nitrogen lone pair, which polarizes the C-Cl bond. In contrast, this compound’s dual electron-withdrawing groups further deactivate the ring, requiring harsher conditions for substitution .
Regioselectivity in Reactions
Nucleophilic Substitution
- This compound : Selective substitution at the 4-position under mild conditions (e.g., alkoxylation with sodium alkoxides) .
- 2,3-Dichloropyridine: Alkoxycarbonylation at 140–160°C yields mono- or diesters depending on temperature, unlike the predictable 4-selectivity of this compound .
Cross-Coupling Reactions
- Suzuki-Miyaura Coupling :
Data Tables
Table 1. Reactivity Comparison of Dichloropyridines
Biological Activity
2,4-Dichloropyridine is a compound that has garnered significant attention in the field of medicinal chemistry due to its diverse biological activities. This article explores its pharmacological properties, mechanisms of action, and potential applications based on recent research findings.
Overview of this compound
This compound is a halogenated derivative of pyridine that exhibits a range of biological activities including antibacterial, antifungal, and anticancer properties. Its structure allows it to interact with various biological targets, making it a valuable compound in drug development.
Antimicrobial Activity
Recent studies have highlighted the antimicrobial efficacy of this compound derivatives against various pathogens. For instance:
- Mycobacterium tuberculosis : Certain derivatives demonstrated significant bactericidal activity against M. tuberculosis, particularly in intracellular environments within human macrophages. The compounds were effective at concentrations below 1 μg/ml, showing potential as new anti-tuberculosis agents .
- Gram-positive and Gram-negative Bacteria : this compound derivatives have shown effectiveness against both Staphylococcus aureus (including MRSA) and Escherichia coli. The mechanisms involved include inhibition of bacterial cell division by disrupting FtsZ polymerization .
Table 1: Antimicrobial Efficacy of this compound Derivatives
Pathogen | Minimum Inhibitory Concentration (MIC) | Activity Type |
---|---|---|
Mycobacterium tuberculosis | <1 μg/ml | Bactericidal |
Staphylococcus aureus | Varies (sub-μg/ml) | Antibacterial |
Escherichia coli | Varies (sub-μg/ml) | Antibacterial |
Anticancer Properties
This compound has also been explored for its anticancer potential. Research indicates:
- Inhibition of Cancer Cell Proliferation : Certain derivatives have shown significant anti-proliferative effects against various cancer cell lines, such as HepG-2 (liver cancer) and MDA-MB-231 (breast cancer). The compounds were tested using standard cytotoxicity assays like MTT and crystal violet assays .
- Mechanisms of Action : The anticancer activity is believed to be mediated through the induction of apoptosis and inhibition of specific signaling pathways involved in tumor growth. For example, some derivatives act as inhibitors of epidermal growth factor receptor (EGFR), which is crucial in many cancers .
Table 2: Anticancer Activity of this compound Derivatives
Cancer Cell Line | IC50 Value (μM) | Mechanism |
---|---|---|
HepG-2 | 10.5 | EGFR inhibition |
MDA-MB-231 | 7.8 | Apoptosis induction |
U87-EGFRvⅢ | 5.0 | Targeting EGFR mutations |
Case Study 1: Resistance Mechanisms in Mycobacterium tuberculosis
A study investigated the resistance mechanisms developed by M. tuberculosis strains exposed to this compound derivatives. Whole-genome sequencing revealed mutations in the mmpR5 gene associated with increased resistance to these compounds. This highlights the need for combination therapies to prevent resistance development .
Case Study 2: Structural Modifications for Enhanced Activity
Research focused on synthesizing new analogs of this compound to enhance its biological activity. Modifications led to improved binding affinities for bacterial targets and increased cytotoxicity against cancer cell lines. These findings suggest that structural optimization can significantly impact the pharmacological profile of pyridine derivatives .
Q & A
Basic Research Questions
Q. What are the most reliable synthetic routes for preparing 2,4-dichloropyridine, and how can regioselectivity be controlled?
Methodological Answer: this compound can be synthesized via direct chlorination of pyridine derivatives or functionalization of pre-chlorinated intermediates. A regioselective approach involves liquid-phase photochlorination, which selectively targets the 2- and 4-positions of pyridine under UV light, achieving >99.0% purity . For functionalization, lithiation using lithium diisopropylamide (LDA) at -78°C in THF, followed by quenching with dry ice, enables carboxylation at the 3-position while retaining the 2,4-dichloro structure . Key factors for regioselectivity include reaction temperature, solvent polarity, and steric effects of directing groups.
Q. How can researchers purify and characterize this compound to ensure high purity for experimental use?
Methodological Answer:
- Purification: Recrystallization from non-polar solvents (e.g., hexane) or vacuum distillation (bp ~189–190°C) removes impurities .
- Characterization:
- GC-MS : Confirms molecular weight (147.98 g/mol) and detects halogen isotopic patterns.
- X-ray Crystallography : Resolves crystal packing and molecular symmetry (e.g., weak aromatic stacking interactions at 3.8 Å separation) .
- NMR : Distinct -NMR signals for H-3 (δ ~8.5 ppm) and H-5 (δ ~7.2 ppm) confirm substitution patterns .
Q. What safety precautions are critical when handling this compound in laboratory settings?
Methodological Answer:
- Personal Protective Equipment (PPE) : Wear nitrile gloves, goggles, and lab coats to avoid skin/eye contact.
- Ventilation : Use fume hoods to mitigate inhalation risks (flash point: 89°C).
- Waste Disposal : Collect halogenated waste separately for incineration to prevent environmental release .
Advanced Research Questions
Q. How do thermodynamic properties influence the stability and reactivity of this compound isomers?
Methodological Answer: The enthalpy of formation () for chloropyridines depends on substituent positions. For this compound, the enthalpy increment for Cl substitution at meta (32.86 ± 1.5 kJ/mol) is lower than ortho (35.96 ± 1.9 kJ/mol), stabilizing the 2,4-isomer over 2,3- or 3,5-analogs . This thermodynamic preference explains the dominance of this compound in synthesis. However, discrepancies in 2,3-dichloropyridine’s experimental vs. estimated suggest unresolved steric or electronic effects, warrantiting remeasurement .
Q. What strategies optimize structure-activity relationships (SAR) for this compound derivatives in pharmacological studies?
Methodological Answer:
- Hydrazide Linker Modification : Replace the hydrazide group with bioisosteres (e.g., amides) to enhance P2X7 receptor binding.
- Hydrophobic Substituents : Introduce polycycloalkyl groups at the R position (e.g., compound 52 ) to improve antagonistic activity (IC = 13 nM in HEK293 cells) .
- In Silico Modeling : Use MarvinSketch or similar tools to predict pKa (e.g., ~1.3 for this compound) and guide substituent selection .
Q. How can electrochemical methods elucidate the redox behavior of this compound in ionic liquids?
Methodological Answer:
- Cyclic Voltammetry (CV) : Measure reduction potentials in aprotic solvents (e.g., [BMIM][NTf]) to identify protonation sites.
- Chronoamperometry : Estimate diffusion coefficients () of this compound derivatives using microdisk electrodes and Shoup-Szabo analysis .
- Simulations : Digitally model voltammetric responses with DigiElch software to correlate electronic structure with redox activity .
Q. How should researchers address contradictions in experimental data, such as anomalous thermochemical values for dichloropyridine isomers?
Methodological Answer:
- Cross-Validation : Replicate measurements using multiple techniques (e.g., combustion calorimetry vs. computational DFT).
- Error Analysis : Quantify uncertainties in enthalpy increments (±1.5–2.2 kJ/mol) .
- Literature Review : Compare with structurally analogous compounds (e.g., 2,6-dichloropyridine) to identify systemic errors .
Properties
IUPAC Name |
2,4-dichloropyridine | |
---|---|---|
Source | PubChem | |
URL | https://pubchem.ncbi.nlm.nih.gov | |
Description | Data deposited in or computed by PubChem | |
InChI |
InChI=1S/C5H3Cl2N/c6-4-1-2-8-5(7)3-4/h1-3H | |
Source | PubChem | |
URL | https://pubchem.ncbi.nlm.nih.gov | |
Description | Data deposited in or computed by PubChem | |
InChI Key |
TYPVHTOETJVYIV-UHFFFAOYSA-N | |
Source | PubChem | |
URL | https://pubchem.ncbi.nlm.nih.gov | |
Description | Data deposited in or computed by PubChem | |
Canonical SMILES |
C1=CN=C(C=C1Cl)Cl | |
Source | PubChem | |
URL | https://pubchem.ncbi.nlm.nih.gov | |
Description | Data deposited in or computed by PubChem | |
Molecular Formula |
C5H3Cl2N | |
Source | PubChem | |
URL | https://pubchem.ncbi.nlm.nih.gov | |
Description | Data deposited in or computed by PubChem | |
DSSTOX Substance ID |
DTXSID20181047 | |
Record name | 2,4-Dichloropyridine | |
Source | EPA DSSTox | |
URL | https://comptox.epa.gov/dashboard/DTXSID20181047 | |
Description | DSSTox provides a high quality public chemistry resource for supporting improved predictive toxicology. | |
Molecular Weight |
147.99 g/mol | |
Source | PubChem | |
URL | https://pubchem.ncbi.nlm.nih.gov | |
Description | Data deposited in or computed by PubChem | |
CAS No. |
26452-80-2 | |
Record name | 2,4-Dichloropyridine | |
Source | CAS Common Chemistry | |
URL | https://commonchemistry.cas.org/detail?cas_rn=26452-80-2 | |
Description | CAS Common Chemistry is an open community resource for accessing chemical information. Nearly 500,000 chemical substances from CAS REGISTRY cover areas of community interest, including common and frequently regulated chemicals, and those relevant to high school and undergraduate chemistry classes. This chemical information, curated by our expert scientists, is provided in alignment with our mission as a division of the American Chemical Society. | |
Explanation | The data from CAS Common Chemistry is provided under a CC-BY-NC 4.0 license, unless otherwise stated. | |
Record name | 2,4-Dichloropyridine | |
Source | ChemIDplus | |
URL | https://pubchem.ncbi.nlm.nih.gov/substance/?source=chemidplus&sourceid=0026452802 | |
Description | ChemIDplus is a free, web search system that provides access to the structure and nomenclature authority files used for the identification of chemical substances cited in National Library of Medicine (NLM) databases, including the TOXNET system. | |
Record name | 2,4-Dichloropyridine | |
Source | EPA DSSTox | |
URL | https://comptox.epa.gov/dashboard/DTXSID20181047 | |
Description | DSSTox provides a high quality public chemistry resource for supporting improved predictive toxicology. | |
Record name | 2,4-dichloropyridine | |
Source | European Chemicals Agency (ECHA) | |
URL | https://echa.europa.eu/substance-information/-/substanceinfo/100.043.364 | |
Description | The European Chemicals Agency (ECHA) is an agency of the European Union which is the driving force among regulatory authorities in implementing the EU's groundbreaking chemicals legislation for the benefit of human health and the environment as well as for innovation and competitiveness. | |
Explanation | Use of the information, documents and data from the ECHA website is subject to the terms and conditions of this Legal Notice, and subject to other binding limitations provided for under applicable law, the information, documents and data made available on the ECHA website may be reproduced, distributed and/or used, totally or in part, for non-commercial purposes provided that ECHA is acknowledged as the source: "Source: European Chemicals Agency, http://echa.europa.eu/". Such acknowledgement must be included in each copy of the material. ECHA permits and encourages organisations and individuals to create links to the ECHA website under the following cumulative conditions: Links can only be made to webpages that provide a link to the Legal Notice page. | |
Retrosynthesis Analysis
AI-Powered Synthesis Planning: Our tool employs the Template_relevance Pistachio, Template_relevance Bkms_metabolic, Template_relevance Pistachio_ringbreaker, Template_relevance Reaxys, Template_relevance Reaxys_biocatalysis model, leveraging a vast database of chemical reactions to predict feasible synthetic routes.
One-Step Synthesis Focus: Specifically designed for one-step synthesis, it provides concise and direct routes for your target compounds, streamlining the synthesis process.
Accurate Predictions: Utilizing the extensive PISTACHIO, BKMS_METABOLIC, PISTACHIO_RINGBREAKER, REAXYS, REAXYS_BIOCATALYSIS database, our tool offers high-accuracy predictions, reflecting the latest in chemical research and data.
Strategy Settings
Precursor scoring | Relevance Heuristic |
---|---|
Min. plausibility | 0.01 |
Model | Template_relevance |
Template Set | Pistachio/Bkms_metabolic/Pistachio_ringbreaker/Reaxys/Reaxys_biocatalysis |
Top-N result to add to graph | 6 |
Feasible Synthetic Routes
Disclaimer and Information on In-Vitro Research Products
Please be aware that all articles and product information presented on BenchChem are intended solely for informational purposes. The products available for purchase on BenchChem are specifically designed for in-vitro studies, which are conducted outside of living organisms. In-vitro studies, derived from the Latin term "in glass," involve experiments performed in controlled laboratory settings using cells or tissues. It is important to note that these products are not categorized as medicines or drugs, and they have not received approval from the FDA for the prevention, treatment, or cure of any medical condition, ailment, or disease. We must emphasize that any form of bodily introduction of these products into humans or animals is strictly prohibited by law. It is essential to adhere to these guidelines to ensure compliance with legal and ethical standards in research and experimentation.