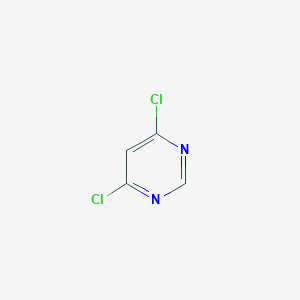
4,6-Dichloropyrimidine
Overview
Description
4,6-Dichloropyrimidine is an organic compound with the molecular formula C4H2Cl2N2. It is a derivative of pyrimidine, a six-membered aromatic heterocycle containing two nitrogen atoms at positions 1 and 3. This compound is characterized by the presence of chlorine atoms at positions 4 and 6 on the pyrimidine ring. It is a yellow-green crystalline solid with a melting point of 65-67°C and a boiling point of 176°C . This compound is widely used in organic synthesis and has significant applications in medicinal chemistry and industrial processes .
Mechanism of Action
Target of Action
4,6-Dichloropyrimidine is a synthetic compound that has been used in various chemical reactions and biological studies . It’s important to note that the compound’s targets can vary depending on the specific context, such as the type of cells or organisms it is interacting with, and the environmental conditions.
Mode of Action
It has been reported that the compound can undergo various chemical reactions, including amination, solvolysis, and condensation processes . These reactions occur under mild and environmentally friendly conditions, influenced by structural factors of the starting pyrimidine and a high concentration of alkoxide ions .
Biochemical Pathways
Pyrimidines, the core structure of this compound, play a crucial role in various biological processes, including dna and rna synthesis
Pharmacokinetics
Understanding these properties is crucial for predicting the compound’s bioavailability, which refers to the extent and rate at which the active moiety (drug or metabolite) enters systemic circulation, thereby accessing the site of action .
Result of Action
It has been reported that 2-amino-4,6-dichloropyrimidine, a related compound, can prevent the replication of certain viruses
Action Environment
Environmental factors can significantly influence the action, efficacy, and stability of a compound like this compound. Factors such as temperature, pH, and the presence of other chemicals can affect the compound’s reactivity and its interactions with biological targets . .
Biochemical Analysis
Biochemical Properties
4,6-Dichloropyrimidine plays a significant role in biochemical reactions. It has been used as a starting reagent for the synthesis of disubstituted pyrimidines by tandem amination and Suzuki-Miyaura cross-coupling
Cellular Effects
It has been found to inhibit immune-activated nitric oxide production . This suggests that it may influence cell function, including impacts on cell signaling pathways, gene expression, and cellular metabolism.
Molecular Mechanism
The molecular mechanism of this compound is complex and not fully elucidated. It is known to participate in various reactions, including the synthesis of N-substituted azacalix4pyrimidines
Dosage Effects in Animal Models
In animal models, 5-fluoro-2-amino-4,6-dichloropyrimidine, a derivative of this compound, has shown inhibitory effects on immune-activated nitric oxide production with an IC50 of 2 µM . The effects of different dosages of this compound itself in animal models have not been extensively studied.
Preparation Methods
Synthetic Routes and Reaction Conditions: 4,6-Dichloropyrimidine can be synthesized through several methods. One common method involves the reaction of 4,6-dihydroxypyrimidine with phosphorus oxychloride (POCl3) and phosphorus pentachloride (PCl5) at temperatures ranging from 50 to 110°C . Another method involves the reaction of 4,6-dihydroxypyrimidine with thionyl chloride (SOCl2) in the presence of a chlorination catalyst . These reactions typically yield high-purity this compound.
Industrial Production Methods: In industrial settings, this compound is produced using large-scale batch processes. The reaction mixture is subjected to reduced pressure distillation to recover phosphorus oxychloride, followed by purification steps to isolate the final product . This method is efficient and environmentally friendly, as it minimizes waste and allows for the recycling of reagents.
Chemical Reactions Analysis
Types of Reactions: 4,6-Dichloropyrimidine undergoes various chemical reactions, including nucleophilic substitution, reduction, and cross-coupling reactions.
Common Reagents and Conditions:
Nucleophilic Substitution: This reaction involves the replacement of chlorine atoms with nucleophiles such as amines or thiols.
Cross-Coupling: Suzuki-Miyaura cross-coupling reactions are commonly used to form biaryl derivatives.
Major Products Formed: The major products formed from these reactions include disubstituted pyrimidines, biarylpyrimidines, and N-substituted azacalix pyrimidines .
Scientific Research Applications
4,6-Dichloropyrimidine has a wide range of applications in scientific research:
Comparison with Similar Compounds
4,6-Dichloropyrimidine can be compared with other similar compounds, such as:
2,4-Dichloropyrimidine: This compound has chlorine atoms at positions 2 and 4 and is used in similar synthetic applications.
4,6-Dihydroxypyrimidine: This compound is a precursor in the synthesis of this compound and has hydroxyl groups at positions 4 and 6.
2,6-Dichloropyridine: This compound has a similar structure but with a pyridine ring instead of a pyrimidine ring.
The uniqueness of this compound lies in its specific substitution pattern, which imparts distinct reactivity and biological activity compared to its analogs.
Properties
IUPAC Name |
4,6-dichloropyrimidine | |
---|---|---|
Source | PubChem | |
URL | https://pubchem.ncbi.nlm.nih.gov | |
Description | Data deposited in or computed by PubChem | |
InChI |
InChI=1S/C4H2Cl2N2/c5-3-1-4(6)8-2-7-3/h1-2H | |
Source | PubChem | |
URL | https://pubchem.ncbi.nlm.nih.gov | |
Description | Data deposited in or computed by PubChem | |
InChI Key |
XJPZKYIHCLDXST-UHFFFAOYSA-N | |
Source | PubChem | |
URL | https://pubchem.ncbi.nlm.nih.gov | |
Description | Data deposited in or computed by PubChem | |
Canonical SMILES |
C1=C(N=CN=C1Cl)Cl | |
Source | PubChem | |
URL | https://pubchem.ncbi.nlm.nih.gov | |
Description | Data deposited in or computed by PubChem | |
Molecular Formula |
C4H2Cl2N2 | |
Source | PubChem | |
URL | https://pubchem.ncbi.nlm.nih.gov | |
Description | Data deposited in or computed by PubChem | |
DSSTOX Substance ID |
DTXSID10152403 | |
Record name | 4,6-Dichloropyrimidine | |
Source | EPA DSSTox | |
URL | https://comptox.epa.gov/dashboard/DTXSID10152403 | |
Description | DSSTox provides a high quality public chemistry resource for supporting improved predictive toxicology. | |
Molecular Weight |
148.98 g/mol | |
Source | PubChem | |
URL | https://pubchem.ncbi.nlm.nih.gov | |
Description | Data deposited in or computed by PubChem | |
CAS No. |
1193-21-1 | |
Record name | 4,6-Dichloropyrimidine | |
Source | CAS Common Chemistry | |
URL | https://commonchemistry.cas.org/detail?cas_rn=1193-21-1 | |
Description | CAS Common Chemistry is an open community resource for accessing chemical information. Nearly 500,000 chemical substances from CAS REGISTRY cover areas of community interest, including common and frequently regulated chemicals, and those relevant to high school and undergraduate chemistry classes. This chemical information, curated by our expert scientists, is provided in alignment with our mission as a division of the American Chemical Society. | |
Explanation | The data from CAS Common Chemistry is provided under a CC-BY-NC 4.0 license, unless otherwise stated. | |
Record name | 4,6-Dichloropyrimidine | |
Source | ChemIDplus | |
URL | https://pubchem.ncbi.nlm.nih.gov/substance/?source=chemidplus&sourceid=0001193211 | |
Description | ChemIDplus is a free, web search system that provides access to the structure and nomenclature authority files used for the identification of chemical substances cited in National Library of Medicine (NLM) databases, including the TOXNET system. | |
Record name | 4,6-Dichloropyrimidine | |
Source | DTP/NCI | |
URL | https://dtp.cancer.gov/dtpstandard/servlet/dwindex?searchtype=NSC&outputformat=html&searchlist=37530 | |
Description | The NCI Development Therapeutics Program (DTP) provides services and resources to the academic and private-sector research communities worldwide to facilitate the discovery and development of new cancer therapeutic agents. | |
Explanation | Unless otherwise indicated, all text within NCI products is free of copyright and may be reused without our permission. Credit the National Cancer Institute as the source. | |
Record name | 4,6-Dichloropyrimidine | |
Source | EPA DSSTox | |
URL | https://comptox.epa.gov/dashboard/DTXSID10152403 | |
Description | DSSTox provides a high quality public chemistry resource for supporting improved predictive toxicology. | |
Record name | 4,6-dichloropyrimidine | |
Source | European Chemicals Agency (ECHA) | |
URL | https://echa.europa.eu/substance-information/-/substanceinfo/100.013.428 | |
Description | The European Chemicals Agency (ECHA) is an agency of the European Union which is the driving force among regulatory authorities in implementing the EU's groundbreaking chemicals legislation for the benefit of human health and the environment as well as for innovation and competitiveness. | |
Explanation | Use of the information, documents and data from the ECHA website is subject to the terms and conditions of this Legal Notice, and subject to other binding limitations provided for under applicable law, the information, documents and data made available on the ECHA website may be reproduced, distributed and/or used, totally or in part, for non-commercial purposes provided that ECHA is acknowledged as the source: "Source: European Chemicals Agency, http://echa.europa.eu/". Such acknowledgement must be included in each copy of the material. ECHA permits and encourages organisations and individuals to create links to the ECHA website under the following cumulative conditions: Links can only be made to webpages that provide a link to the Legal Notice page. | |
Record name | 4,6-Dichloropyrimidine | |
Source | FDA Global Substance Registration System (GSRS) | |
URL | https://gsrs.ncats.nih.gov/ginas/app/beta/substances/TTE9SA8H44 | |
Description | The FDA Global Substance Registration System (GSRS) enables the efficient and accurate exchange of information on what substances are in regulated products. Instead of relying on names, which vary across regulatory domains, countries, and regions, the GSRS knowledge base makes it possible for substances to be defined by standardized, scientific descriptions. | |
Explanation | Unless otherwise noted, the contents of the FDA website (www.fda.gov), both text and graphics, are not copyrighted. They are in the public domain and may be republished, reprinted and otherwise used freely by anyone without the need to obtain permission from FDA. Credit to the U.S. Food and Drug Administration as the source is appreciated but not required. | |
Retrosynthesis Analysis
AI-Powered Synthesis Planning: Our tool employs the Template_relevance Pistachio, Template_relevance Bkms_metabolic, Template_relevance Pistachio_ringbreaker, Template_relevance Reaxys, Template_relevance Reaxys_biocatalysis model, leveraging a vast database of chemical reactions to predict feasible synthetic routes.
One-Step Synthesis Focus: Specifically designed for one-step synthesis, it provides concise and direct routes for your target compounds, streamlining the synthesis process.
Accurate Predictions: Utilizing the extensive PISTACHIO, BKMS_METABOLIC, PISTACHIO_RINGBREAKER, REAXYS, REAXYS_BIOCATALYSIS database, our tool offers high-accuracy predictions, reflecting the latest in chemical research and data.
Strategy Settings
Precursor scoring | Relevance Heuristic |
---|---|
Min. plausibility | 0.01 |
Model | Template_relevance |
Template Set | Pistachio/Bkms_metabolic/Pistachio_ringbreaker/Reaxys/Reaxys_biocatalysis |
Top-N result to add to graph | 6 |
Feasible Synthetic Routes
Q1: What is the molecular formula and weight of 4,6-Dichloropyrimidine?
A1: this compound has the molecular formula C4H2Cl2N2 and a molecular weight of 148.98 g/mol. []
Q2: What spectroscopic data is available for this compound?
A2: this compound has been characterized by various spectroscopic methods including 1H NMR, 13C NMR, HRMS, and IR. [] Additionally, its gas-phase molecular structure has been determined by electron diffraction (GED). []
Q3: What are the common starting materials for synthesizing this compound?
A3: this compound is commonly synthesized from either 4,6-dihydroxypyrimidine [, , , , , , ] or 4-chloro-6-methoxypyrimidine. [, ]
Q4: What are some challenges in the traditional synthesis of this compound and how are they addressed?
A4: Traditional methods using phosphorus oxychloride generate phosphorus-containing byproducts, posing environmental concerns. Alternative approaches utilize phosgene [, , , , ] or sulfur-containing chlorinating agents in the presence of phosphorus compounds [, ] to circumvent this issue. Another challenge is the use of organic bases which require tedious recovery and recycling. Some methods avoid organic bases altogether [, ] while others employ easily recoverable bases like pyridine. []
Q5: Are there any "green" synthesis methods for this compound?
A5: Yes, a "green" synthesis method has been reported that avoids highly toxic reactants and reduces waste by using dichlorophosphoric acid for chlorination and eliminating the need for solvents. []
Q6: What are the main applications of this compound?
A6: this compound serves as a versatile building block in organic synthesis, particularly for preparing various pyrimidine derivatives with applications in pharmaceuticals and agricultural chemicals. [, , , ]
Q7: How does the structure of this compound contribute to its reactivity?
A7: The two chlorine atoms at the 4 and 6 positions of the pyrimidine ring are susceptible to nucleophilic aromatic substitution (SNAr) reactions, making it a valuable scaffold for introducing diverse substituents. [, ]
Q8: Can you give examples of specific reactions and products involving this compound?
A8: this compound reacts with 2-cyanomethylquinoline to yield H-chelates that can act as "intramolecular energy transfer" systems. [, ] It can also be utilized in Suzuki cross-coupling reactions with aryl/heteroaryl boronic acids to produce novel pyrimidine analogs. [] Additionally, it reacts with glycine esters to form pyrrolo[2,3-d]pyrimidine and pyrido[2,3-d]pyrimidine derivatives. []
Q9: What role does this compound play in the synthesis of Dasatinib?
A9: In the synthesis of Dasatinib, a tyrosine kinase inhibitor, this compound is crucial for introducing the 2-methylpyrimidinyl moiety through a nucleophilic substitution reaction. []
Q10: Have computational methods been applied to study this compound?
A10: Yes, Density Functional Theory (DFT) calculations have been employed to investigate the reactivity descriptors and electronic properties of this compound derivatives, providing insights into their stability and potential applications. [] Additionally, ab initio calculations have been used to determine its gas-phase molecular structure and understand the effects of chlorination on the ring geometry. []
Q11: What is known about the crystal structure of this compound?
A11: this compound exists in at least two polymorphic forms. The first, reported in 1948, crystallizes in the monoclinic space group P21/a. [, ] A second monoclinic polymorph, crystallizing in the space group C2/c, was reported later. [] In both polymorphs, the molecule is planar, and the crystal structures are stabilized by N–H⋯N intermolecular hydrogen bonds. []
Q12: Are there any environmental concerns associated with this compound?
A13: While this compound itself hasn't been extensively studied for environmental impact, its production often involves hazardous reagents like phosphorus oxychloride, which can lead to phosphorus-containing waste. [] Therefore, environmentally friendly synthetic alternatives are being explored. [, , , , , ]
Disclaimer and Information on In-Vitro Research Products
Please be aware that all articles and product information presented on BenchChem are intended solely for informational purposes. The products available for purchase on BenchChem are specifically designed for in-vitro studies, which are conducted outside of living organisms. In-vitro studies, derived from the Latin term "in glass," involve experiments performed in controlled laboratory settings using cells or tissues. It is important to note that these products are not categorized as medicines or drugs, and they have not received approval from the FDA for the prevention, treatment, or cure of any medical condition, ailment, or disease. We must emphasize that any form of bodily introduction of these products into humans or animals is strictly prohibited by law. It is essential to adhere to these guidelines to ensure compliance with legal and ethical standards in research and experimentation.