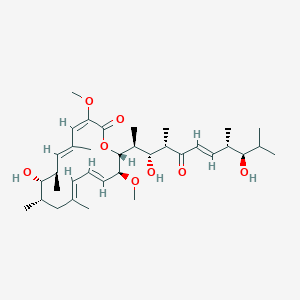
Bafilomycin D
Overview
Description
The compound Bafilomycin D is a complex organic molecule with a unique structure. This compound is characterized by multiple double bonds, hydroxyl groups, and methoxy groups, making it a subject of interest in various fields of scientific research.
Mechanism of Action
Target of Action
Bafilomycin D primarily targets the vacuolar-type H±ATPase (V-ATPase) enzyme . V-ATPase is a membrane-spanning proton pump that acidifies either the extracellular environment or intracellular organelles such as the lysosome of animal cells or the vacuole of plants and fungi .
Mode of Action
This compound shares the same mode of action as Bafilomycin A1 . It acts as a specific inhibitor of V-ATPases , preventing the re-acidification of synaptic vesicles once they have undergone exocytosis . This interaction with its targets leads to significant changes in cellular processes .
Biochemical Pathways
The inhibition of V-ATPase by this compound affects various biochemical pathways. V-ATPase plays a crucial role in many physiological processes, including membrane trafficking, autophagy, apoptosis, bone resorption, and sperm maturation . By inhibiting V-ATPase, this compound can disrupt these processes, leading to various downstream effects .
Result of Action
The inhibition of V-ATPase by this compound leads to significant changes in cellular processes. It can prevent the re-acidification of synaptic vesicles, leading to alterations in neurotransmission . Additionally, it can disrupt autophagy, a cellular degradation pathway that delivers organelles or protein to the lysosome .
Action Environment
The action of this compound can be influenced by various environmental factors. For instance, the pH of the environment can affect the activity of V-ATPase and, consequently, the efficacy of this compound . .
Biochemical Analysis
Biochemical Properties
Bafilomycin D plays a crucial role in biochemical reactions by inhibiting the vacuolar-type H±ATPase (V-ATPase) enzyme. This enzyme is responsible for acidifying intracellular organelles such as lysosomes and endosomes. By inhibiting V-ATPase, this compound disrupts the acidification process, which is essential for various cellular functions, including protein degradation and receptor-mediated endocytosis . This compound interacts with the V-ATPase enzyme by binding to its membrane-spanning proton pump, thereby inhibiting its activity and preventing the acidification of intracellular compartments .
Cellular Effects
This compound has significant effects on various types of cells and cellular processes. It influences cell function by disrupting the acidification of intracellular organelles, which is essential for processes such as protein degradation, autophagy, and receptor-mediated endocytosis . By inhibiting V-ATPase, this compound affects cell signaling pathways, gene expression, and cellular metabolism. For example, it has been shown to induce autophagy by preventing the fusion of autophagosomes with lysosomes, leading to the accumulation of autophagic vacuoles . Additionally, this compound can influence gene expression by altering the pH of intracellular compartments, which affects the activity of pH-sensitive transcription factors .
Molecular Mechanism
The molecular mechanism of this compound involves its interaction with the V-ATPase enzyme. This compound binds to the membrane-spanning proton pump of V-ATPase, inhibiting its activity and preventing the acidification of intracellular organelles . This inhibition disrupts various cellular processes that rely on acidic environments, such as protein degradation, autophagy, and receptor-mediated endocytosis. Additionally, this compound can affect gene expression by altering the pH of intracellular compartments, which influences the activity of pH-sensitive transcription factors .
Temporal Effects in Laboratory Settings
In laboratory settings, the effects of this compound can change over time. The stability and degradation of this compound are important factors that influence its long-term effects on cellular function. Studies have shown that this compound is relatively stable under laboratory conditions, but its activity can decrease over time due to degradation . Long-term exposure to this compound can lead to sustained inhibition of V-ATPase, resulting in prolonged disruption of cellular processes such as autophagy and protein degradation . Additionally, the effects of this compound on cellular function can vary depending on the duration of exposure and the concentration used in experiments .
Dosage Effects in Animal Models
The effects of this compound vary with different dosages in animal models. At low doses, this compound can effectively inhibit V-ATPase activity and disrupt cellular processes such as autophagy and protein degradation . At high doses, this compound can have toxic or adverse effects on cells and tissues . For example, high doses of this compound can lead to excessive accumulation of autophagic vacuoles, resulting in cell death . Additionally, threshold effects have been observed in studies, where a certain concentration of this compound is required to achieve significant inhibition of V-ATPase activity and disruption of cellular processes .
Metabolic Pathways
This compound is involved in various metabolic pathways, including those related to the biosynthesis of its polyketide backbone. In the bafilomycin biosynthetic gene cluster of Streptomyces griseus DSM 2608, five polyketide synthase (PKS) genes coding 12 PKS modules are responsible for the biosynthesis of the bafilomycin polyketide backbone . These PKS modules utilize methoxymalonyl-acyl carrier protein (ACP) as an extender unit to incorporate methoxy groups into the polyketide structure . The biosynthetic pathway of methoxymalonyl-ACP involves several enzymatic steps, including the conversion of 1,3-bis-phosphoglycerate to methoxymalonyl-ACP by the action of specific enzymes encoded by the bafilomycin biosynthetic gene cluster .
Transport and Distribution
This compound is transported and distributed within cells and tissues through various mechanisms. It can interact with transporters and binding proteins that facilitate its uptake and distribution within cells . Once inside the cell, this compound can accumulate in specific intracellular compartments, such as lysosomes and endosomes, where it exerts its inhibitory effects on V-ATPase . The localization and accumulation of this compound within these compartments are essential for its activity and function .
Subcellular Localization
The subcellular localization of this compound is primarily within acidic intracellular compartments, such as lysosomes and endosomes . This localization is crucial for its inhibitory effects on V-ATPase and the subsequent disruption of cellular processes that rely on acidic environments . This compound can be directed to specific compartments or organelles through targeting signals or post-translational modifications that influence its localization and activity .
Preparation Methods
Synthetic Routes and Reaction Conditions
The synthesis of this compound involves multiple steps, including the formation of the core structure and the introduction of various functional groups. The synthetic route typically starts with the preparation of the core cyclohexadeca structure, followed by the introduction of hydroxyl and methoxy groups through selective reactions. Common reagents used in these reactions include strong acids and bases, oxidizing agents, and reducing agents.
Industrial Production Methods
Industrial production of this compound may involve large-scale synthesis using automated reactors and continuous flow systems. The reaction conditions are optimized to ensure high yield and purity of the final product. Techniques such as chromatography and crystallization are employed to purify the compound.
Chemical Reactions Analysis
Types of Reactions
This compound undergoes various types of chemical reactions, including:
Oxidation: The hydroxyl groups can be oxidized to form ketones or aldehydes.
Reduction: The double bonds can be reduced to single bonds using hydrogenation reactions.
Substitution: The methoxy groups can be substituted with other functional groups through nucleophilic substitution reactions.
Common Reagents and Conditions
Oxidation: Common oxidizing agents include potassium permanganate (KMnO4) and chromium trioxide (CrO3).
Reduction: Hydrogen gas (H2) in the presence of a palladium catalyst (Pd/C) is commonly used for hydrogenation.
Substitution: Nucleophiles such as sodium methoxide (NaOCH3) are used for substitution reactions.
Major Products
The major products formed from these reactions depend on the specific conditions and reagents used. For example, oxidation of the hydroxyl groups can lead to the formation of ketones, while reduction of the double bonds results in the formation of saturated hydrocarbons.
Scientific Research Applications
This compound has a wide range of applications in scientific research, including:
Chemistry: Used as a building block for the synthesis of more complex molecules.
Biology: Studied for its potential biological activity and interactions with biomolecules.
Medicine: Investigated for its potential therapeutic properties, including anti-inflammatory and anticancer activities.
Industry: Used in the development of new materials and chemical processes.
Comparison with Similar Compounds
Similar Compounds
4,4’-Difluorobenzophenone: An organic compound used as a precursor to high-performance polymers.
Noble Gas Compounds: Compounds involving noble gases, which are generally unreactive but can form stable compounds under certain conditions.
Uniqueness
The uniqueness of this compound lies in its complex structure and the presence of multiple functional groups, which confer a wide range of chemical reactivity and potential biological activity. Its ability to undergo various chemical reactions and interact with different molecular targets makes it a valuable compound for research and industrial applications.
Properties
CAS No. |
98813-13-9 |
---|---|
Molecular Formula |
C35H56O8 |
Molecular Weight |
604.8 g/mol |
IUPAC Name |
(3Z,5E,7R,8S,9S,11E,13E,15S,16R)-16-[(E,2S,3R,4S,8S,9R)-3,9-dihydroxy-4,8,10-trimethyl-5-oxoundec-6-en-2-yl]-8-hydroxy-3,15-dimethoxy-5,7,9,11-tetramethyl-1-oxacyclohexadeca-3,5,11,13-tetraen-2-one |
InChI |
InChI=1S/C35H56O8/c1-20(2)31(37)23(5)15-16-28(36)26(8)33(39)27(9)34-29(41-10)14-12-13-21(3)17-24(6)32(38)25(7)18-22(4)19-30(42-11)35(40)43-34/h12-16,18-20,23-27,29,31-34,37-39H,17H2,1-11H3/b14-12+,16-15+,21-13+,22-18+,30-19-/t23-,24-,25+,26+,27-,29-,31+,32-,33-,34+/m0/s1 |
InChI Key |
ZKOTUWJMGBWBEO-DTOYTSOJSA-N |
SMILES |
CC1CC(=CC=CC(C(OC(=O)C(=CC(=CC(C1O)C)C)OC)C(C)C(C(C)C(=O)C=CC(C)C(C(C)C)O)O)OC)C |
Isomeric SMILES |
C[C@H]1C/C(=C/C=C/[C@@H]([C@H](OC(=O)/C(=C/C(=C/[C@H]([C@H]1O)C)/C)/OC)[C@@H](C)[C@H]([C@H](C)C(=O)/C=C/[C@H](C)[C@@H](C(C)C)O)O)OC)/C |
Canonical SMILES |
CC1CC(=CC=CC(C(OC(=O)C(=CC(=CC(C1O)C)C)OC)C(C)C(C(C)C(=O)C=CC(C)C(C(C)C)O)O)OC)C |
Appearance |
White powder |
Synonyms |
(3Z,5E,7R,8S,9S,11E,13E,15S,16R)-16-[(1S,2R,3S,5E,7S,8R)-2,8-dihydroxy-1,3,7,9-tetramethyl-4-oxo-5-decen-1-yl]-8-hydroxy-3,15-dimethoxy-5,7,9,11-tetramethyl-oxacyclohexadeca-3,5,11,13-tetraen-2-one |
Origin of Product |
United States |
Retrosynthesis Analysis
AI-Powered Synthesis Planning: Our tool employs the Template_relevance Pistachio, Template_relevance Bkms_metabolic, Template_relevance Pistachio_ringbreaker, Template_relevance Reaxys, Template_relevance Reaxys_biocatalysis model, leveraging a vast database of chemical reactions to predict feasible synthetic routes.
One-Step Synthesis Focus: Specifically designed for one-step synthesis, it provides concise and direct routes for your target compounds, streamlining the synthesis process.
Accurate Predictions: Utilizing the extensive PISTACHIO, BKMS_METABOLIC, PISTACHIO_RINGBREAKER, REAXYS, REAXYS_BIOCATALYSIS database, our tool offers high-accuracy predictions, reflecting the latest in chemical research and data.
Strategy Settings
Precursor scoring | Relevance Heuristic |
---|---|
Min. plausibility | 0.01 |
Model | Template_relevance |
Template Set | Pistachio/Bkms_metabolic/Pistachio_ringbreaker/Reaxys/Reaxys_biocatalysis |
Top-N result to add to graph | 6 |
Feasible Synthetic Routes
Disclaimer and Information on In-Vitro Research Products
Please be aware that all articles and product information presented on BenchChem are intended solely for informational purposes. The products available for purchase on BenchChem are specifically designed for in-vitro studies, which are conducted outside of living organisms. In-vitro studies, derived from the Latin term "in glass," involve experiments performed in controlled laboratory settings using cells or tissues. It is important to note that these products are not categorized as medicines or drugs, and they have not received approval from the FDA for the prevention, treatment, or cure of any medical condition, ailment, or disease. We must emphasize that any form of bodily introduction of these products into humans or animals is strictly prohibited by law. It is essential to adhere to these guidelines to ensure compliance with legal and ethical standards in research and experimentation.