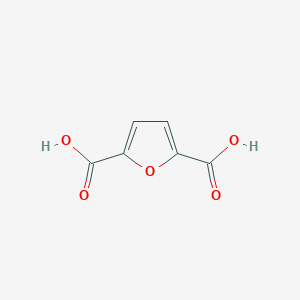
2,5-Furandicarboxylic acid
Overview
Description
2,5-Furandicarboxylic acid (FDCA) is a five-carbon dicarboxylic acid, which is a derivative of furan and a key intermediate in the production of polymers and other materials. FDCA is a versatile chemical compound and has been used in a wide range of applications, including in the production of plastics and other materials, in chemical synthesis, and in medical research. FDCA has also been used as a precursor for the synthesis of other compounds, such as pharmaceuticals and agrochemicals, and as a building block in the synthesis of polymers.
Scientific Research Applications
Monomer and Polymer Synthesis
FDCA is a versatile furanic building block for chemical and polymer applications . It is used as a precursor for monomer and polymer synthesis . However, the classical FDCA production methodology has significant drawbacks that hinder industrial-scale production .
Sustainable Polymers
FDCA is used in the production of sustainable polymers . The development of sustainable processes for renewable bioplastics for packaging offers the potential to replace around 2% of oil consumption .
Biobased Monomers
FDCA is used in the production of biobased monomers . The U.S. Department of Energy designated FDCA as one of the “Top Value-Added Chemicals from Biomass” .
Furan-based Materials
FDCA is used in the production of furan-based materials . The various potential applications arise from these furan-based materials .
Biosynthesis from 5-hydroxymethylfurfural (HMF)
FDCA can be biosynthesized from HMF via a bi-enzymatic cascade system using bacterial laccase and fungal alcohol oxidase . This biocatalysis approach is cost-effective and eco-friendly .
Production of Polyethylene Furanoate (PEF)
FDCA, along with biobased ethylene glycol, can be used to make sustainable polyethylene furanoate (PEF), a polyester that has properties similar to those of the widely used polyethylene terephthalate (PET) .
Alternative to Terephthalic Acid
FDCA and PEF are now considered as an alternative to terephthalic acid and PET, which are produced from fossil sources . FDCA can be obtained by dehydration of polysaccharides and biomass feedstock to HMF with its following catalytic oxidation .
Biomass-based Building Blocks
FDCA is considered one of the “Top 12” promising biomass-based building blocks . It exhibits excellent thermochemical properties with biodegradability, excellent gas barrier performance, recyclability, and extended mechanical properties .
Mechanism of Action
Target of Action
2,5-Furandicarboxylic acid (FDCA) is a versatile furanic building block for chemical and polymer applications . It is a strong complexing agent that chelates ions such as Ca 2+, Cu 2+, and Pb 2+ . It is also used in medicine to remove kidney stones .
Mode of Action
FDCA interacts with its targets primarily through oxidation. It oxidizes the intermediates of conventional oxidation without the formation of CO 2 and CO, thus increasing the yield of FDCA . This oxidation process is facilitated by enzymes such as bacterial laccase from Bacillus pumilus and alcohol oxidase from Colletotrichum gloeosporioides .
Biochemical Pathways
The production of FDCA generally starts with the oxidation of 5-Hydroxymethylfurfural (HMF) . HMF can be transformed into various high value-added chemicals, such as furan and furan derivatives, carboxylic acids, lactones and lactam, diol and alkanes . In particular, the hydrolysis of cellulose from lignocellulosic biomass generates sugars, which can be used for HMF production after dehydration under acidic conditions .
Pharmacokinetics
Information on the pharmacokinetics of FDCA, including its absorption, distribution, metabolism, and excretion (ADME) properties, is currently limited. It is known that fdca is soluble in water and dmso , which may influence its bioavailability.
Result of Action
The primary result of FDCA’s action is the production of bio-based polymers and other furan-based materials . These materials have potential applications in various industries, including the packaging industry .
Action Environment
The action of FDCA can be influenced by various environmental factors. For instance, the yield of FDCA can be affected by the reaction conditions, such as temperature and pH . Additionally, the transformation efficiency of HMF to FDCA can be boosted by certain enzymes .
properties
IUPAC Name |
furan-2,5-dicarboxylic acid | |
---|---|---|
Source | PubChem | |
URL | https://pubchem.ncbi.nlm.nih.gov | |
Description | Data deposited in or computed by PubChem | |
InChI |
InChI=1S/C6H4O5/c7-5(8)3-1-2-4(11-3)6(9)10/h1-2H,(H,7,8)(H,9,10) | |
Source | PubChem | |
URL | https://pubchem.ncbi.nlm.nih.gov | |
Description | Data deposited in or computed by PubChem | |
InChI Key |
CHTHALBTIRVDBM-UHFFFAOYSA-N | |
Source | PubChem | |
URL | https://pubchem.ncbi.nlm.nih.gov | |
Description | Data deposited in or computed by PubChem | |
Canonical SMILES |
C1=C(OC(=C1)C(=O)O)C(=O)O | |
Source | PubChem | |
URL | https://pubchem.ncbi.nlm.nih.gov | |
Description | Data deposited in or computed by PubChem | |
Molecular Formula |
C6H4O5 | |
Source | PubChem | |
URL | https://pubchem.ncbi.nlm.nih.gov | |
Description | Data deposited in or computed by PubChem | |
DSSTOX Substance ID |
DTXSID0062927 | |
Record name | 2,5-Furandicarboxylic acid | |
Source | EPA DSSTox | |
URL | https://comptox.epa.gov/dashboard/DTXSID0062927 | |
Description | DSSTox provides a high quality public chemistry resource for supporting improved predictive toxicology. | |
Molecular Weight |
156.09 g/mol | |
Source | PubChem | |
URL | https://pubchem.ncbi.nlm.nih.gov | |
Description | Data deposited in or computed by PubChem | |
Physical Description |
Solid | |
Record name | 2,5-Furandicarboxylic acid | |
Source | Human Metabolome Database (HMDB) | |
URL | http://www.hmdb.ca/metabolites/HMDB0004812 | |
Description | The Human Metabolome Database (HMDB) is a freely available electronic database containing detailed information about small molecule metabolites found in the human body. | |
Explanation | HMDB is offered to the public as a freely available resource. Use and re-distribution of the data, in whole or in part, for commercial purposes requires explicit permission of the authors and explicit acknowledgment of the source material (HMDB) and the original publication (see the HMDB citing page). We ask that users who download significant portions of the database cite the HMDB paper in any resulting publications. | |
Solubility |
1 mg/mL at 18 °C | |
Record name | 2,5-Furandicarboxylic acid | |
Source | Human Metabolome Database (HMDB) | |
URL | http://www.hmdb.ca/metabolites/HMDB0004812 | |
Description | The Human Metabolome Database (HMDB) is a freely available electronic database containing detailed information about small molecule metabolites found in the human body. | |
Explanation | HMDB is offered to the public as a freely available resource. Use and re-distribution of the data, in whole or in part, for commercial purposes requires explicit permission of the authors and explicit acknowledgment of the source material (HMDB) and the original publication (see the HMDB citing page). We ask that users who download significant portions of the database cite the HMDB paper in any resulting publications. | |
Product Name |
2,5-Furandicarboxylic acid | |
CAS RN |
3238-40-2 | |
Record name | 2,5-Furandicarboxylic acid | |
Source | CAS Common Chemistry | |
URL | https://commonchemistry.cas.org/detail?cas_rn=3238-40-2 | |
Description | CAS Common Chemistry is an open community resource for accessing chemical information. Nearly 500,000 chemical substances from CAS REGISTRY cover areas of community interest, including common and frequently regulated chemicals, and those relevant to high school and undergraduate chemistry classes. This chemical information, curated by our expert scientists, is provided in alignment with our mission as a division of the American Chemical Society. | |
Explanation | The data from CAS Common Chemistry is provided under a CC-BY-NC 4.0 license, unless otherwise stated. | |
Record name | 2,5-Furandicarboxylic acid | |
Source | ChemIDplus | |
URL | https://pubchem.ncbi.nlm.nih.gov/substance/?source=chemidplus&sourceid=0003238402 | |
Description | ChemIDplus is a free, web search system that provides access to the structure and nomenclature authority files used for the identification of chemical substances cited in National Library of Medicine (NLM) databases, including the TOXNET system. | |
Record name | 2,5-Furandicarboxylic acid | |
Source | DTP/NCI | |
URL | https://dtp.cancer.gov/dtpstandard/servlet/dwindex?searchtype=NSC&outputformat=html&searchlist=40740 | |
Description | The NCI Development Therapeutics Program (DTP) provides services and resources to the academic and private-sector research communities worldwide to facilitate the discovery and development of new cancer therapeutic agents. | |
Explanation | Unless otherwise indicated, all text within NCI products is free of copyright and may be reused without our permission. Credit the National Cancer Institute as the source. | |
Record name | 2,5-Furandicarboxylic acid | |
Source | EPA Chemicals under the TSCA | |
URL | https://www.epa.gov/chemicals-under-tsca | |
Description | EPA Chemicals under the Toxic Substances Control Act (TSCA) collection contains information on chemicals and their regulations under TSCA, including non-confidential content from the TSCA Chemical Substance Inventory and Chemical Data Reporting. | |
Record name | 2,5-Furandicarboxylic acid | |
Source | EPA DSSTox | |
URL | https://comptox.epa.gov/dashboard/DTXSID0062927 | |
Description | DSSTox provides a high quality public chemistry resource for supporting improved predictive toxicology. | |
Record name | Furan-2,5-dicarboxylic acid | |
Source | European Chemicals Agency (ECHA) | |
URL | https://echa.europa.eu/substance-information/-/substanceinfo/100.019.819 | |
Description | The European Chemicals Agency (ECHA) is an agency of the European Union which is the driving force among regulatory authorities in implementing the EU's groundbreaking chemicals legislation for the benefit of human health and the environment as well as for innovation and competitiveness. | |
Explanation | Use of the information, documents and data from the ECHA website is subject to the terms and conditions of this Legal Notice, and subject to other binding limitations provided for under applicable law, the information, documents and data made available on the ECHA website may be reproduced, distributed and/or used, totally or in part, for non-commercial purposes provided that ECHA is acknowledged as the source: "Source: European Chemicals Agency, http://echa.europa.eu/". Such acknowledgement must be included in each copy of the material. ECHA permits and encourages organisations and individuals to create links to the ECHA website under the following cumulative conditions: Links can only be made to webpages that provide a link to the Legal Notice page. | |
Record name | 2,5-FURANDICARBOXYLIC ACID | |
Source | FDA Global Substance Registration System (GSRS) | |
URL | https://gsrs.ncats.nih.gov/ginas/app/beta/substances/73C4JJ695C | |
Description | The FDA Global Substance Registration System (GSRS) enables the efficient and accurate exchange of information on what substances are in regulated products. Instead of relying on names, which vary across regulatory domains, countries, and regions, the GSRS knowledge base makes it possible for substances to be defined by standardized, scientific descriptions. | |
Explanation | Unless otherwise noted, the contents of the FDA website (www.fda.gov), both text and graphics, are not copyrighted. They are in the public domain and may be republished, reprinted and otherwise used freely by anyone without the need to obtain permission from FDA. Credit to the U.S. Food and Drug Administration as the source is appreciated but not required. | |
Record name | 2,5-Furandicarboxylic acid | |
Source | Human Metabolome Database (HMDB) | |
URL | http://www.hmdb.ca/metabolites/HMDB0004812 | |
Description | The Human Metabolome Database (HMDB) is a freely available electronic database containing detailed information about small molecule metabolites found in the human body. | |
Explanation | HMDB is offered to the public as a freely available resource. Use and re-distribution of the data, in whole or in part, for commercial purposes requires explicit permission of the authors and explicit acknowledgment of the source material (HMDB) and the original publication (see the HMDB citing page). We ask that users who download significant portions of the database cite the HMDB paper in any resulting publications. | |
Melting Point |
342 °C | |
Record name | 2,5-Furandicarboxylic acid | |
Source | Human Metabolome Database (HMDB) | |
URL | http://www.hmdb.ca/metabolites/HMDB0004812 | |
Description | The Human Metabolome Database (HMDB) is a freely available electronic database containing detailed information about small molecule metabolites found in the human body. | |
Explanation | HMDB is offered to the public as a freely available resource. Use and re-distribution of the data, in whole or in part, for commercial purposes requires explicit permission of the authors and explicit acknowledgment of the source material (HMDB) and the original publication (see the HMDB citing page). We ask that users who download significant portions of the database cite the HMDB paper in any resulting publications. | |
Retrosynthesis Analysis
AI-Powered Synthesis Planning: Our tool employs the Template_relevance Pistachio, Template_relevance Bkms_metabolic, Template_relevance Pistachio_ringbreaker, Template_relevance Reaxys, Template_relevance Reaxys_biocatalysis model, leveraging a vast database of chemical reactions to predict feasible synthetic routes.
One-Step Synthesis Focus: Specifically designed for one-step synthesis, it provides concise and direct routes for your target compounds, streamlining the synthesis process.
Accurate Predictions: Utilizing the extensive PISTACHIO, BKMS_METABOLIC, PISTACHIO_RINGBREAKER, REAXYS, REAXYS_BIOCATALYSIS database, our tool offers high-accuracy predictions, reflecting the latest in chemical research and data.
Strategy Settings
Precursor scoring | Relevance Heuristic |
---|---|
Min. plausibility | 0.01 |
Model | Template_relevance |
Template Set | Pistachio/Bkms_metabolic/Pistachio_ringbreaker/Reaxys/Reaxys_biocatalysis |
Top-N result to add to graph | 6 |
Feasible Synthetic Routes
Q & A
Q1: What are the main challenges in producing FDCA on an industrial scale?
A1: The classical method for FDCA production, involving the oxidation of 5-hydroxymethylfurfural (HMF), faces drawbacks that hinder large-scale production. These challenges include:
Q2: What alternative methods are being explored to synthesize FDCA more efficiently and sustainably?
A2: Researchers are investigating various innovative approaches for FDCA synthesis, including:
- Use of non-noble metal catalysts: Employing catalysts based on manganese oxide [], cobalt-bismuth binary oxide [], or cobalt boride/g-C3N4 nanosheets [] offers a cost-effective alternative to precious metal catalysts.
- Electrocatalytic oxidation: This method utilizes electricity to drive the oxidation of HMF to FDCA, potentially reducing the reliance on chemical oxidants [, , ].
- Biocatalytic conversion: Utilizing enzymes like aryl alcohol oxidase, peroxygenase, and galactose oxidase offers a milder and more environmentally friendly approach [].
- Direct conversion from fructose: This strategy simplifies the process by eliminating the need for separate HMF production [].
Q3: Can you elaborate on the use of non-noble metal catalysts in FDCA synthesis?
A3: Non-noble metal catalysts, such as manganese oxide [] or cobalt-bismuth binary oxide [], are being actively researched for their potential to replace expensive noble metal catalysts in FDCA production. These catalysts have shown promising activity and selectivity towards FDCA while being significantly more cost-effective.
Q4: How does the choice of catalyst affect the efficiency of FDCA synthesis?
A4: The catalyst plays a critical role in determining the reaction pathway, yield, and selectivity of FDCA synthesis []. Different catalysts, such as gold nanoparticles on various supports [, ], ruthenium supported on zirconia [], or cobalt boride [], exhibit different activities and selectivities towards FDCA. For instance, gold catalysts have shown high selectivity towards FDCA [], while silver catalysts exhibit a preference for producing 5-hydroxymethyl-2-furancarboxylic acid (HFCA) [].
Q5: Can FDCA be produced directly from fructose, bypassing the HMF intermediate?
A5: Yes, researchers have developed processes for the direct conversion of fructose to FDCA []. This approach utilizes a combined dehydration-oxidation strategy, often employing a bifunctional catalyst that can facilitate both reactions in one pot. This direct conversion eliminates the need for HMF isolation and purification, simplifying the process and potentially improving its overall efficiency.
Q6: What makes FDCA a suitable replacement for terephthalic acid in polyester production?
A6: FDCA shares a structural similarity with terephthalic acid, making it a promising substitute in the production of polyesters [, ]. FDCA-based polyesters, such as polyethylene furanoate (PEF), exhibit comparable or even superior properties to their petroleum-based counterparts, including enhanced thermal stability, improved barrier properties (e.g., reduced gas permeability) [, , , ], and potential for biodegradability [].
Q7: What specific properties of FDCA-based polyesters make them attractive for packaging applications?
A7: FDCA-based polyesters, like PEF, possess several advantageous properties for packaging, including:
- Excellent barrier properties: PEF exhibits significantly lower permeability to oxygen and carbon dioxide compared to traditional PET, making it ideal for preserving the freshness and shelf life of food and beverages [, , ].
- Improved thermal stability: The higher glass transition temperature of PEF allows for its use in hot-fill applications, expanding its potential applications in food packaging [, ].
- Potential for biodegradability: Research suggests that FDCA-based polyesters may be more susceptible to biodegradation than conventional PET, offering a more sustainable end-of-life solution [].
Q8: Beyond packaging, what other applications are being explored for FDCA-based polymers?
A8: The unique properties of FDCA-based polymers have spurred research into diverse applications beyond packaging, including:
- Textiles: The incorporation of FDCA into polyesters for textile fibers can enhance their thermal and mechanical properties [, ].
- Coatings: FDCA-based resins exhibit potential for use in high-performance coatings due to their good adhesion, thermal stability, and resistance to chemicals [, ].
- Thermosets: FDCA can be used to synthesize epoxy resins with high biomass content, potentially leading to more sustainable alternatives to traditional epoxy resins [, , ].
Q9: How does the incorporation of other monomers affect the properties of FDCA-based copolymers?
A9: The properties of FDCA-based copolymers can be tailored by incorporating different comonomers into the polymer backbone [, , , ]. For example, adding 1,4-cyclohexanedimethanol (CHDM) to poly(butylene 2,5-furandicarboxylate) (PBF) increases its glass transition temperature (Tg), thermal stability, and influences its crystallinity []. Similarly, incorporating 2,2,4,4-tetramethyl-1,3-cyclobutanediol (CBDO) into PEF, PPF, and PBF results in copolyesters with increased Tg, improved barrier properties, and enhanced transparency [].
Q10: How does the polymerization method influence the properties of the resulting FDCA-based polymer?
A10: The polymerization method significantly impacts the molecular weight, sequence distribution, and ultimately, the properties of FDCA-based polymers. Different polymerization techniques, such as direct esterification [, ], transesterification [], and ring-opening polymerization [], can lead to variations in the final polymer characteristics.
Q11: How does the use of FDCA contribute to a more sustainable plastics industry?
A11: FDCA's derivation from renewable biomass sources, such as sugars obtained from plants [], positions it as a sustainable alternative to petroleum-based terephthalic acid. This shift towards bio-based monomers helps reduce the reliance on fossil fuels and promotes a circular economy by utilizing renewable resources.
Q12: Are there any environmental concerns associated with the production or disposal of FDCA-based materials?
A12: While FDCA-based materials offer a promising route towards sustainable plastics, some environmental considerations remain:
Q13: How are computational methods being applied in FDCA research?
A13: Computational chemistry plays a crucial role in understanding and optimizing FDCA synthesis and its applications:
- Catalyst design: Density functional theory (DFT) calculations can be used to model and predict the catalytic activity and selectivity of various catalysts for HMF oxidation to FDCA [].
- Polymer property prediction: Molecular dynamics (MD) simulations can predict the thermal, mechanical, and barrier properties of FDCA-based polymers, aiding in the design of materials with desired characteristics [].
Disclaimer and Information on In-Vitro Research Products
Please be aware that all articles and product information presented on BenchChem are intended solely for informational purposes. The products available for purchase on BenchChem are specifically designed for in-vitro studies, which are conducted outside of living organisms. In-vitro studies, derived from the Latin term "in glass," involve experiments performed in controlled laboratory settings using cells or tissues. It is important to note that these products are not categorized as medicines or drugs, and they have not received approval from the FDA for the prevention, treatment, or cure of any medical condition, ailment, or disease. We must emphasize that any form of bodily introduction of these products into humans or animals is strictly prohibited by law. It is essential to adhere to these guidelines to ensure compliance with legal and ethical standards in research and experimentation.