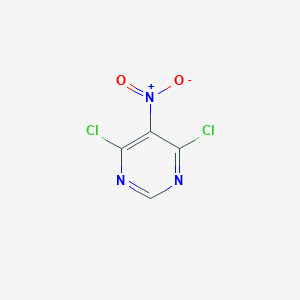
4,6-Dichloro-5-nitropyrimidine
Overview
Description
4,6-Dichloro-5-nitropyrimidine is a chemical compound with the molecular formula C4HCl2N3O2 and a molecular weight of 193.98 g/mol . It is a white to yellow crystalline solid that is primarily used as an intermediate in organic synthesis . This compound is known for its reactivity due to the presence of both chloro and nitro groups on the pyrimidine ring, making it a valuable building block in the synthesis of various pharmaceuticals and agrochemicals .
Preparation Methods
Synthetic Routes and Reaction Conditions
4,6-Dichloro-5-nitropyrimidine can be synthesized through several methods. One common method involves the reaction of 4,6-dihydroxy-5-nitropyrimidine with phosphorus oxychloride (POCl3) under reflux conditions . The reaction typically proceeds as follows:
Starting Material: 4,6-dihydroxy-5-nitropyrimidine
Reagent: Phosphorus oxychloride (POCl3)
Conditions: Reflux
Product: this compound
Another method involves the nitration of 4,6-dichloropyrimidine using a mixture of nitric acid and sulfuric acid . The reaction conditions are as follows:
Starting Material: 4,6-dichloropyrimidine
Reagents: Nitric acid (HNO3) and sulfuric acid (H2SO4)
Conditions: Controlled temperature
Industrial Production Methods
Industrial production of this compound often involves large-scale synthesis using the aforementioned methods. The process is optimized for high yield and purity, with careful control of reaction conditions to ensure consistency and safety . The use of continuous flow reactors and automated systems can further enhance the efficiency and scalability of the production process.
Chemical Reactions Analysis
Types of Reactions
4,6-Dichloro-5-nitropyrimidine undergoes various types of chemical reactions, including:
Nucleophilic Substitution: The chloro groups can be replaced by nucleophiles such as amines, thiols, and alkoxides.
Reduction: The nitro group can be reduced to an amino group using reducing agents like zinc and acetic acid.
Cyclization: It can participate in cyclization reactions to form fused heterocyclic compounds.
Common Reagents and Conditions
Nucleophilic Substitution: Reagents such as primary amines (e.g., aniline) and conditions like room temperature in the presence of a base (e.g., triethylamine) are commonly used.
Reduction: Zinc and acetic acid are typical reagents for the reduction of the nitro group.
Cyclization: Cyclization reactions often require specific catalysts and controlled temperatures.
Major Products Formed
Nucleophilic Substitution: Substituted pyrimidines with various functional groups depending on the nucleophile used.
Reduction: 4,6-Dichloro-5-aminopyrimidine.
Scientific Research Applications
4,6-Dichloro-5-nitropyrimidine has a wide range of applications in scientific research:
Mechanism of Action
The mechanism of action of 4,6-Dichloro-5-nitropyrimidine is primarily based on its ability to undergo nucleophilic substitution reactions. The chloro groups are highly reactive and can be replaced by various nucleophiles, leading to the formation of new compounds with different biological activities . The nitro group can also be reduced to an amino group, which can further participate in various biochemical pathways .
Comparison with Similar Compounds
Similar Compounds
Uniqueness
4,6-Dichloro-5-nitropyrimidine is unique due to the presence of both chloro and nitro groups on the pyrimidine ring, which imparts high reactivity and versatility in chemical synthesis . This dual functionality allows it to participate in a wide range of reactions, making it a valuable intermediate in the synthesis of various complex molecules .
Properties
IUPAC Name |
4,6-dichloro-5-nitropyrimidine | |
---|---|---|
Source | PubChem | |
URL | https://pubchem.ncbi.nlm.nih.gov | |
Description | Data deposited in or computed by PubChem | |
InChI |
InChI=1S/C4HCl2N3O2/c5-3-2(9(10)11)4(6)8-1-7-3/h1H | |
Source | PubChem | |
URL | https://pubchem.ncbi.nlm.nih.gov | |
Description | Data deposited in or computed by PubChem | |
InChI Key |
HCTISZQLTGAYOX-UHFFFAOYSA-N | |
Source | PubChem | |
URL | https://pubchem.ncbi.nlm.nih.gov | |
Description | Data deposited in or computed by PubChem | |
Canonical SMILES |
C1=NC(=C(C(=N1)Cl)[N+](=O)[O-])Cl | |
Source | PubChem | |
URL | https://pubchem.ncbi.nlm.nih.gov | |
Description | Data deposited in or computed by PubChem | |
Molecular Formula |
C4HCl2N3O2 | |
Source | PubChem | |
URL | https://pubchem.ncbi.nlm.nih.gov | |
Description | Data deposited in or computed by PubChem | |
DSSTOX Substance ID |
DTXSID9063409 | |
Record name | 4,6-Dichloro-5-nitropyrimidine | |
Source | EPA DSSTox | |
URL | https://comptox.epa.gov/dashboard/DTXSID9063409 | |
Description | DSSTox provides a high quality public chemistry resource for supporting improved predictive toxicology. | |
Molecular Weight |
193.97 g/mol | |
Source | PubChem | |
URL | https://pubchem.ncbi.nlm.nih.gov | |
Description | Data deposited in or computed by PubChem | |
CAS No. |
4316-93-2 | |
Record name | 4,6-Dichloro-5-nitropyrimidine | |
Source | CAS Common Chemistry | |
URL | https://commonchemistry.cas.org/detail?cas_rn=4316-93-2 | |
Description | CAS Common Chemistry is an open community resource for accessing chemical information. Nearly 500,000 chemical substances from CAS REGISTRY cover areas of community interest, including common and frequently regulated chemicals, and those relevant to high school and undergraduate chemistry classes. This chemical information, curated by our expert scientists, is provided in alignment with our mission as a division of the American Chemical Society. | |
Explanation | The data from CAS Common Chemistry is provided under a CC-BY-NC 4.0 license, unless otherwise stated. | |
Record name | Pyrimidine, 4,6-dichloro-5-nitro- | |
Source | ChemIDplus | |
URL | https://pubchem.ncbi.nlm.nih.gov/substance/?source=chemidplus&sourceid=0004316932 | |
Description | ChemIDplus is a free, web search system that provides access to the structure and nomenclature authority files used for the identification of chemical substances cited in National Library of Medicine (NLM) databases, including the TOXNET system. | |
Record name | 4,6-Dichloro-5-nitropyrimidine | |
Source | DTP/NCI | |
URL | https://dtp.cancer.gov/dtpstandard/servlet/dwindex?searchtype=NSC&outputformat=html&searchlist=89693 | |
Description | The NCI Development Therapeutics Program (DTP) provides services and resources to the academic and private-sector research communities worldwide to facilitate the discovery and development of new cancer therapeutic agents. | |
Explanation | Unless otherwise indicated, all text within NCI products is free of copyright and may be reused without our permission. Credit the National Cancer Institute as the source. | |
Record name | Pyrimidine, 4,6-dichloro-5-nitro- | |
Source | EPA Chemicals under the TSCA | |
URL | https://www.epa.gov/chemicals-under-tsca | |
Description | EPA Chemicals under the Toxic Substances Control Act (TSCA) collection contains information on chemicals and their regulations under TSCA, including non-confidential content from the TSCA Chemical Substance Inventory and Chemical Data Reporting. | |
Record name | 4,6-Dichloro-5-nitropyrimidine | |
Source | EPA DSSTox | |
URL | https://comptox.epa.gov/dashboard/DTXSID9063409 | |
Description | DSSTox provides a high quality public chemistry resource for supporting improved predictive toxicology. | |
Record name | 4,6-dichloro-5-nitro-pyridine | |
Source | European Chemicals Agency (ECHA) | |
URL | https://echa.europa.eu/substance-information/-/substanceinfo/100.022.128 | |
Description | The European Chemicals Agency (ECHA) is an agency of the European Union which is the driving force among regulatory authorities in implementing the EU's groundbreaking chemicals legislation for the benefit of human health and the environment as well as for innovation and competitiveness. | |
Explanation | Use of the information, documents and data from the ECHA website is subject to the terms and conditions of this Legal Notice, and subject to other binding limitations provided for under applicable law, the information, documents and data made available on the ECHA website may be reproduced, distributed and/or used, totally or in part, for non-commercial purposes provided that ECHA is acknowledged as the source: "Source: European Chemicals Agency, http://echa.europa.eu/". Such acknowledgement must be included in each copy of the material. ECHA permits and encourages organisations and individuals to create links to the ECHA website under the following cumulative conditions: Links can only be made to webpages that provide a link to the Legal Notice page. | |
Retrosynthesis Analysis
AI-Powered Synthesis Planning: Our tool employs the Template_relevance Pistachio, Template_relevance Bkms_metabolic, Template_relevance Pistachio_ringbreaker, Template_relevance Reaxys, Template_relevance Reaxys_biocatalysis model, leveraging a vast database of chemical reactions to predict feasible synthetic routes.
One-Step Synthesis Focus: Specifically designed for one-step synthesis, it provides concise and direct routes for your target compounds, streamlining the synthesis process.
Accurate Predictions: Utilizing the extensive PISTACHIO, BKMS_METABOLIC, PISTACHIO_RINGBREAKER, REAXYS, REAXYS_BIOCATALYSIS database, our tool offers high-accuracy predictions, reflecting the latest in chemical research and data.
Strategy Settings
Precursor scoring | Relevance Heuristic |
---|---|
Min. plausibility | 0.01 |
Model | Template_relevance |
Template Set | Pistachio/Bkms_metabolic/Pistachio_ringbreaker/Reaxys/Reaxys_biocatalysis |
Top-N result to add to graph | 6 |
Feasible Synthetic Routes
Disclaimer and Information on In-Vitro Research Products
Please be aware that all articles and product information presented on BenchChem are intended solely for informational purposes. The products available for purchase on BenchChem are specifically designed for in-vitro studies, which are conducted outside of living organisms. In-vitro studies, derived from the Latin term "in glass," involve experiments performed in controlled laboratory settings using cells or tissues. It is important to note that these products are not categorized as medicines or drugs, and they have not received approval from the FDA for the prevention, treatment, or cure of any medical condition, ailment, or disease. We must emphasize that any form of bodily introduction of these products into humans or animals is strictly prohibited by law. It is essential to adhere to these guidelines to ensure compliance with legal and ethical standards in research and experimentation.