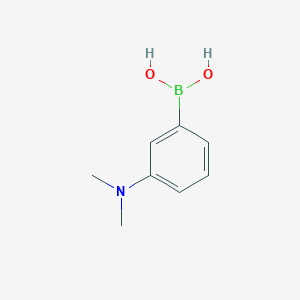
3-(N,N-Dimethylamino)phenylboronic acid
Overview
Description
3-(N,N-Dimethylamino)phenylboronic acid is a chemical compound with the empirical formula C8H12BNO2 . It is used as a reactant in the synthesis of various protein effectors, including modulators of survival motor neuron protein, glucokinase activators, and aryl ethers for use as Bacillus anthracis enoyl-ACP reductase inhibitors .
Synthesis Analysis
The synthesis of borinic acid derivatives, including 3-(N,N-Dimethylamino)phenylboronic acid, has seen recent advances . The main strategies to build up borinic acids rely either on the addition of organometallic reagents to boranes or the reaction of triarylboranes with a ligand .Molecular Structure Analysis
The molecular weight of 3-(N,N-Dimethylamino)phenylboronic acid is 165.00 . The linear formula of this compound is C8H12BNO2 .Chemical Reactions Analysis
This compound is involved in the synthesis of different protein effectors, including modulators of survival motor neuron protein, glucokinase activators, and aryl ethers for use as Bacillus anthracis enoyl-ACP reductase inhibitors . It is also used in the synthesis of thiourea-functionalized paracyclophanes .Physical And Chemical Properties Analysis
The melting point of 3-(N,N-Dimethylamino)phenylboronic acid is between 178-190 °C . It has an assay of ≥95% .Scientific Research Applications
Chemical Properties
“3-(N,N-Dimethylamino)phenylboronic acid” has a molecular weight of 164.9974 g/mol and a melting point of 142-144 °C . Its chemical formula is C₈H₁₂BNO₂ .
Sugar Chemosensor
This compound has been used in the creation of a new sugar chemosensor, known as a boron–rhodamine-containing carboxylic acid (BRhoC) . The borinate group of BRhoC reacts with a diol moiety of sugar to form a cyclic ester, which induces a change in the absorbance and fluorescence spectra .
Glucose Detection
The boron–rhodamine-containing carboxylic acid (BRhoC) has been used to detect D-glucose . The binding constants (K) were determined to be 2.3 × 10² M⁻¹ for D-fructose (Fru) and 3.1 M⁻¹ for D-glucose .
Modulators of Survival Motor Neuron Protein
The compound has been used in the synthesis of modulators of survival motor neuron protein .
Glucokinase Activators
It has also been used in the synthesis of glucokinase activators .
Aryl Ethers for Bacillus Anthracis Enoyl-ACP Reductase Inhibitors
This compound has been used in the synthesis of aryl ethers for use as Bacillus anthracis enoyl-ACP reductase inhibitors .
Thiourea-Functionalized Paracyclophanes
It has been involved in the synthesis of thiourea-functionalized paracyclophanes .
Safety and Hazards
This compound is classified as an irritant and can cause harm to the respiratory system . It is recommended to avoid dust formation, breathing mist, gas or vapours, and contact with skin and eyes. Personal protective equipment such as a dust mask type N95 (US), eyeshields, and gloves are recommended .
Mechanism of Action
Target of Action
3-(N,N-Dimethylamino)phenylboronic acid is a reactant involved in the synthesis of different protein effectors . It has been used in the creation of modulators of survival motor neuron proteins, glucokinase activators, and aryl ethers for use as Bacillus anthracis enoyl-ACP reductase inhibitors .
Mode of Action
The compound is a weak acid and can participate in acid-base reactions . Its amine group can lose a proton, making it reactive . It can also undergo organic synthesis reactions, such as forming nucleophilic addition products with aldehydes .
Biochemical Pathways
It has been used in the synthesis of modulators of survival motor neuron proteins, glucokinase activators, and aryl ethers for use as bacillus anthracis enoyl-acp reductase inhibitors . These suggest that it may have an impact on the pathways related to motor neuron survival, glucose metabolism, and bacterial fatty acid synthesis.
Pharmacokinetics
Its solubility in water and organic solvents like ether, ethanol, and dimethylformamide suggests that it may have good bioavailability .
Result of Action
The molecular and cellular effects of 3-(N,N-Dimethylamino)phenylboronic acid’s action depend on the specific context of its use. In the synthesis of protein effectors, it can contribute to the modulation of survival motor neuron proteins, activation of glucokinase, and inhibition of Bacillus anthracis enoyl-ACP reductase .
Action Environment
The action, efficacy, and stability of 3-(N,N-Dimethylamino)phenylboronic acid can be influenced by environmental factors. For instance, it is sensitive to heat and may decompose to produce toxic gases at high temperatures . It should be stored in a dry, well-ventilated place, away from sources of ignition . The compound is also sensitive to moisture and may contain varying amounts of anhydride .
properties
IUPAC Name |
[3-(dimethylamino)phenyl]boronic acid | |
---|---|---|
Source | PubChem | |
URL | https://pubchem.ncbi.nlm.nih.gov | |
Description | Data deposited in or computed by PubChem | |
InChI |
InChI=1S/C8H12BNO2/c1-10(2)8-5-3-4-7(6-8)9(11)12/h3-6,11-12H,1-2H3 | |
Source | PubChem | |
URL | https://pubchem.ncbi.nlm.nih.gov | |
Description | Data deposited in or computed by PubChem | |
InChI Key |
YZQQHZXHCXAJAV-UHFFFAOYSA-N | |
Source | PubChem | |
URL | https://pubchem.ncbi.nlm.nih.gov | |
Description | Data deposited in or computed by PubChem | |
Canonical SMILES |
B(C1=CC(=CC=C1)N(C)C)(O)O | |
Source | PubChem | |
URL | https://pubchem.ncbi.nlm.nih.gov | |
Description | Data deposited in or computed by PubChem | |
Molecular Formula |
C8H12BNO2 | |
Source | PubChem | |
URL | https://pubchem.ncbi.nlm.nih.gov | |
Description | Data deposited in or computed by PubChem | |
DSSTOX Substance ID |
DTXSID90376286 | |
Record name | 3-(N,N-Dimethylamino)phenylboronic acid | |
Source | EPA DSSTox | |
URL | https://comptox.epa.gov/dashboard/DTXSID90376286 | |
Description | DSSTox provides a high quality public chemistry resource for supporting improved predictive toxicology. | |
Molecular Weight |
165.00 g/mol | |
Source | PubChem | |
URL | https://pubchem.ncbi.nlm.nih.gov | |
Description | Data deposited in or computed by PubChem | |
Product Name |
3-(N,N-Dimethylamino)phenylboronic acid | |
CAS RN |
178752-79-9 | |
Record name | 3-(N,N-Dimethylamino)phenylboronic acid | |
Source | EPA DSSTox | |
URL | https://comptox.epa.gov/dashboard/DTXSID90376286 | |
Description | DSSTox provides a high quality public chemistry resource for supporting improved predictive toxicology. | |
Record name | 3-(Dimethylamino)benzeneboronic acid | |
Source | European Chemicals Agency (ECHA) | |
URL | https://echa.europa.eu/information-on-chemicals | |
Description | The European Chemicals Agency (ECHA) is an agency of the European Union which is the driving force among regulatory authorities in implementing the EU's groundbreaking chemicals legislation for the benefit of human health and the environment as well as for innovation and competitiveness. | |
Explanation | Use of the information, documents and data from the ECHA website is subject to the terms and conditions of this Legal Notice, and subject to other binding limitations provided for under applicable law, the information, documents and data made available on the ECHA website may be reproduced, distributed and/or used, totally or in part, for non-commercial purposes provided that ECHA is acknowledged as the source: "Source: European Chemicals Agency, http://echa.europa.eu/". Such acknowledgement must be included in each copy of the material. ECHA permits and encourages organisations and individuals to create links to the ECHA website under the following cumulative conditions: Links can only be made to webpages that provide a link to the Legal Notice page. | |
Retrosynthesis Analysis
AI-Powered Synthesis Planning: Our tool employs the Template_relevance Pistachio, Template_relevance Bkms_metabolic, Template_relevance Pistachio_ringbreaker, Template_relevance Reaxys, Template_relevance Reaxys_biocatalysis model, leveraging a vast database of chemical reactions to predict feasible synthetic routes.
One-Step Synthesis Focus: Specifically designed for one-step synthesis, it provides concise and direct routes for your target compounds, streamlining the synthesis process.
Accurate Predictions: Utilizing the extensive PISTACHIO, BKMS_METABOLIC, PISTACHIO_RINGBREAKER, REAXYS, REAXYS_BIOCATALYSIS database, our tool offers high-accuracy predictions, reflecting the latest in chemical research and data.
Strategy Settings
Precursor scoring | Relevance Heuristic |
---|---|
Min. plausibility | 0.01 |
Model | Template_relevance |
Template Set | Pistachio/Bkms_metabolic/Pistachio_ringbreaker/Reaxys/Reaxys_biocatalysis |
Top-N result to add to graph | 6 |
Feasible Synthetic Routes
Disclaimer and Information on In-Vitro Research Products
Please be aware that all articles and product information presented on BenchChem are intended solely for informational purposes. The products available for purchase on BenchChem are specifically designed for in-vitro studies, which are conducted outside of living organisms. In-vitro studies, derived from the Latin term "in glass," involve experiments performed in controlled laboratory settings using cells or tissues. It is important to note that these products are not categorized as medicines or drugs, and they have not received approval from the FDA for the prevention, treatment, or cure of any medical condition, ailment, or disease. We must emphasize that any form of bodily introduction of these products into humans or animals is strictly prohibited by law. It is essential to adhere to these guidelines to ensure compliance with legal and ethical standards in research and experimentation.