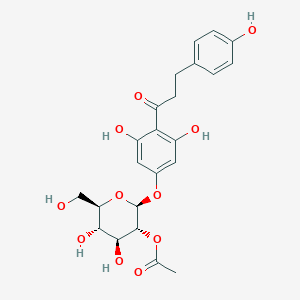
Trilobatin 2''-acetate
Overview
Description
Trilobatin 2’'-acetate is a sweet dihydrochalcone glucoside derived from the leaves of Lithocarpus pachyphyllus. It is known for its intense sweetness and minimal caloric value, making it an attractive natural sweetener. This compound has gained attention due to its potential health benefits, including anti-inflammatory and glucose-lowering effects .
Mechanism of Action
Target of Action
Trilobatin 2/‘/’-acetate, a natural dihydrochalcone compound, has been found to primarily target the Nrf2/ARE and IRS-1/GLUT2 signaling pathways . These pathways play vital roles in oxidative stress and insulin signaling transduction, which are crucial in the pathogenesis of type 2 diabetes mellitus (T2DM) .
Mode of Action
Trilobatin 2/‘/’-acetate interacts with its targets by activating the Nrf2/ARE signaling pathway and regulating the insulin signaling transduction pathway . It promotes Nrf2 translocation from the cytoplasm to the nucleus, increases the protein expressions of HO-1, NQO-1, and GLUT-2, and the phosphorylation levels of Akt and GSK-3 βSer 9. It also decreases the protein expressions of keap1 and the phosphorylation levels of IRS-1 Ser 307 and GSK-3 βTyr 216 .
Biochemical Pathways
The compound affects the Nrf2/ARE and IRS-1/GLUT2 signaling pathways . Activation of the Nrf2/ARE pathway leads to the transcription of antioxidant response element-dependent genes, which encode detoxifying enzymes and antioxidant proteins. This results in the reduction of reactive oxygen species and enhancement of antioxidant enzymes activities . The regulation of the IRS-1/GLUT2 pathway improves insulin resistance and promotes tolerances to exogenous glucose and insulin .
Pharmacokinetics
It’s known that the compound is derived from the leaves of lithocarpus polystachyus , suggesting that it might be absorbed through ingestion
Result of Action
Trilobatin 2/‘/’-acetate has been shown to exhibit anti-T2DM effects in KK-Ay mice . It significantly reduces high fasting blood glucose levels and insulin resistance, promotes tolerances to exogenous glucose and insulin, and regulates abnormal parameters of lipid metabolism . It also ameliorates the pancreatic islet morphology and increases the insulin expression of the islet .
Action Environment
The action of Trilobatin 2/‘/’-acetate can be influenced by various environmental factors. For instance, the compound’s efficacy can be affected by the physiological state of the organism, such as the presence of oxidative stress or insulin resistance . Additionally, the compound’s stability might be influenced by storage conditions . .
Biochemical Analysis
Biochemical Properties
Trilobatin 2/‘/’-acetate interacts with various enzymes and proteins. For instance, it has been found to be efficiently catalyzed by Phloretin Glycosyltransferase2 (PGT2) to form 4′-o-glycosylation of phloretin . This interaction plays a crucial role in the biosynthesis of Trilobatin 2/‘/’-acetate .
Cellular Effects
Trilobatin 2/‘/’-acetate has been shown to have significant effects on various types of cells and cellular processes. For example, it has been found to increase glucose consumption, glycogen content, and the activity of hexokinase (HK) and pyruvate kinase (PK) in insulin-resistant HepG2 cells . It also reduces the content of reactive oxygen species and enhances antioxidant enzyme activities .
Molecular Mechanism
The molecular mechanism of Trilobatin 2/‘/’-acetate involves its interaction with various biomolecules. It has been found to regulate glucose metabolism through the PI3K/Akt/GSK-3β and Nrf2/Keap-1 signaling pathways, improving insulin resistance and reducing oxidative stress .
Temporal Effects in Laboratory Settings
While specific studies on the temporal effects of Trilobatin 2/‘/’-acetate in laboratory settings are limited, it has been shown to have significant effects on glucose metabolism and oxidative stress over time .
Dosage Effects in Animal Models
In animal models, Trilobatin 2/‘/’-acetate has been shown to significantly reduce the high fasting blood glucose level and insulin resistance in KK-Ay diabetic mice when administered at different doses (10, 50, and 100 mg/kg/d) for 4 weeks .
Metabolic Pathways
Trilobatin 2/‘/’-acetate is involved in various metabolic pathways. It has been found to ameliorate glycogen synthesis via the phosphatidylinositol 3-kinase (PI3K)/protein kinase B (Akt)/glycogen synthase kinase-3β (GSK-3β) signaling pathway .
Transport and Distribution
It has been found to bind to Bovine Serum Albumin (BSA), which may aid in its transport and distribution .
Subcellular Localization
It has been found to promote Nrf2 that was translocated from cytoplasm to nucleus , suggesting that it may have effects on the activity or function of specific compartments or organelles.
Preparation Methods
Synthetic Routes and Reaction Conditions
The biosynthesis of trilobatin 2’‘-acetate involves the glycosylation of phloretin by the enzyme phloretin glycosyltransferase2 (PGT2). This enzyme catalyzes the 4’-O-glycosylation of phloretin to produce trilobatin. The reaction conditions typically involve the use of transient expression systems in Nicotiana benthamiana, where genes encoding chalcone synthase, double bond reductase, and PGT2 are introduced to reconstitute the biosynthetic pathway .
Industrial Production Methods
Industrial production of trilobatin 2’'-acetate can be achieved through biopharming and metabolic engineering in yeast. This approach allows for the large-scale production of the compound by optimizing the expression of key enzymes involved in its biosynthesis. The use of transgenic plants overexpressing PGT2 has also been explored to produce high concentrations of trilobatin in young leaves .
Chemical Reactions Analysis
Types of Reactions
Trilobatin 2’'-acetate undergoes various chemical reactions, including:
Oxidation: This reaction involves the addition of oxygen or the removal of hydrogen, leading to the formation of oxidized products.
Reduction: This reaction involves the addition of hydrogen or the removal of oxygen, resulting in reduced products.
Substitution: This reaction involves the replacement of one functional group with another, leading to the formation of substituted products.
Common Reagents and Conditions
Common reagents used in the reactions of trilobatin 2’'-acetate include oxidizing agents like hydrogen peroxide, reducing agents like sodium borohydride, and various catalysts to facilitate substitution reactions. The reaction conditions typically involve controlled temperatures and pH levels to ensure optimal yields .
Major Products Formed
The major products formed from the reactions of trilobatin 2’'-acetate include oxidized derivatives, reduced forms, and substituted compounds with modified functional groups .
Scientific Research Applications
Trilobatin 2’'-acetate has a wide range of scientific research applications, including:
Chemistry: It is used as a model compound to study glycosylation reactions and the synthesis of sweeteners.
Biology: It is investigated for its antioxidant and anti-inflammatory properties, as well as its potential to modulate glucose metabolism.
Medicine: Trilobatin 2’'-acetate is explored for its potential therapeutic effects in managing diabetes and other metabolic disorders.
Industry: It is used as a natural sweetener in food and beverage products, offering a healthier alternative to traditional sugars .
Comparison with Similar Compounds
Trilobatin 2’'-acetate can be compared with other similar compounds, such as:
Phloridzin: A bitter positional isomer of trilobatin found in domesticated apple leaves.
Trilobatin: The non-acetylated form of trilobatin 2’'-acetate, which also exhibits sweetening properties and health benefits.
Trilobatin 2’'-acetate stands out due to its unique combination of sweetness, minimal caloric value, and potential health benefits, making it a valuable compound for various applications.
Biological Activity
Trilobatin 2''-acetate, a dihydrochalcone glycoside derived from Lithocarpus pachyphyllus, has garnered attention for its diverse biological activities, particularly its antioxidative properties and potential therapeutic effects in metabolic disorders such as Type 2 Diabetes Mellitus (T2DM). This article synthesizes current research findings on the biological activity of this compound, highlighting its mechanisms of action, efficacy in various models, and implications for future therapeutic applications.
Chemical Structure and Properties
This compound is characterized by its sweet taste and low caloric content, making it a candidate for natural food additives. Its molecular formula is with a molecular weight of 478.45 g/mol. The compound's structure contributes to its bioactivity, particularly in antioxidant and anti-diabetic mechanisms.
Antioxidant Activity
Research has demonstrated that this compound exhibits significant antioxidant properties. A study reported the following IC50 values for lipid peroxidation inhibitory activity:
Compound | IC50 (µM) |
---|---|
This compound | 261 |
Phloridzin | 28 |
Trilobatin | 88 |
Additionally, the compound increased superoxide dismutase (SOD) and glutathione peroxidase (GSH-Px) activities with EC50 values of 575 µM and 717 µM respectively, indicating its potential to mitigate oxidative stress in biological systems .
Anti-Diabetic Effects
This compound has been investigated for its anti-diabetic effects, particularly in animal models of T2DM. In a study involving KK-Ay diabetic mice, Trilobatin significantly reduced fasting blood glucose levels and improved insulin resistance. The treatment with Trilobatin led to:
- Enhanced tolerance to exogenous glucose and insulin.
- Reduction in reactive oxygen species (ROS) levels.
- Increased activities of antioxidant enzymes such as catalase, glutathione peroxidase, and superoxide dismutase.
- Regulation of lipid metabolism parameters including triglycerides and cholesterol levels .
The underlying mechanism appears to involve the activation of the Nrf2/ARE signaling pathway, which is crucial for cellular antioxidant responses. This pathway facilitates the translocation of Nrf2 from the cytoplasm to the nucleus, leading to the upregulation of genes involved in antioxidant defense .
Case Studies
- KK-Ay Diabetic Mice Model : In this model, mice treated with Trilobatin showed significant improvements in pancreatic islet morphology and insulin expression compared to untreated controls. The study highlighted that Trilobatin's ability to modulate insulin signaling pathways could provide a novel approach for managing T2DM .
- Neuroprotective Effects : Previous studies have indicated that Trilobatin exhibits neuroprotective effects due to its antioxidative profile. This suggests potential applications beyond metabolic disorders, possibly extending into neurodegenerative conditions where oxidative stress plays a critical role .
Properties
IUPAC Name |
[(2S,3R,4S,5S,6R)-2-[3,5-dihydroxy-4-[3-(4-hydroxyphenyl)propanoyl]phenoxy]-4,5-dihydroxy-6-(hydroxymethyl)oxan-3-yl] acetate | |
---|---|---|
Source | PubChem | |
URL | https://pubchem.ncbi.nlm.nih.gov | |
Description | Data deposited in or computed by PubChem | |
InChI |
InChI=1S/C23H26O11/c1-11(25)32-22-21(31)20(30)18(10-24)34-23(22)33-14-8-16(28)19(17(29)9-14)15(27)7-4-12-2-5-13(26)6-3-12/h2-3,5-6,8-9,18,20-24,26,28-31H,4,7,10H2,1H3/t18-,20-,21+,22-,23-/m1/s1 | |
Source | PubChem | |
URL | https://pubchem.ncbi.nlm.nih.gov | |
Description | Data deposited in or computed by PubChem | |
InChI Key |
QIEAJYJXGQAYRU-DODNOZFWSA-N | |
Source | PubChem | |
URL | https://pubchem.ncbi.nlm.nih.gov | |
Description | Data deposited in or computed by PubChem | |
Canonical SMILES |
CC(=O)OC1C(C(C(OC1OC2=CC(=C(C(=C2)O)C(=O)CCC3=CC=C(C=C3)O)O)CO)O)O | |
Source | PubChem | |
URL | https://pubchem.ncbi.nlm.nih.gov | |
Description | Data deposited in or computed by PubChem | |
Isomeric SMILES |
CC(=O)O[C@@H]1[C@H]([C@@H]([C@H](O[C@H]1OC2=CC(=C(C(=C2)O)C(=O)CCC3=CC=C(C=C3)O)O)CO)O)O | |
Source | PubChem | |
URL | https://pubchem.ncbi.nlm.nih.gov | |
Description | Data deposited in or computed by PubChem | |
Molecular Formula |
C23H26O11 | |
Source | PubChem | |
URL | https://pubchem.ncbi.nlm.nih.gov | |
Description | Data deposited in or computed by PubChem | |
Molecular Weight |
478.4 g/mol | |
Source | PubChem | |
URL | https://pubchem.ncbi.nlm.nih.gov | |
Description | Data deposited in or computed by PubChem | |
Retrosynthesis Analysis
AI-Powered Synthesis Planning: Our tool employs the Template_relevance Pistachio, Template_relevance Bkms_metabolic, Template_relevance Pistachio_ringbreaker, Template_relevance Reaxys, Template_relevance Reaxys_biocatalysis model, leveraging a vast database of chemical reactions to predict feasible synthetic routes.
One-Step Synthesis Focus: Specifically designed for one-step synthesis, it provides concise and direct routes for your target compounds, streamlining the synthesis process.
Accurate Predictions: Utilizing the extensive PISTACHIO, BKMS_METABOLIC, PISTACHIO_RINGBREAKER, REAXYS, REAXYS_BIOCATALYSIS database, our tool offers high-accuracy predictions, reflecting the latest in chemical research and data.
Strategy Settings
Precursor scoring | Relevance Heuristic |
---|---|
Min. plausibility | 0.01 |
Model | Template_relevance |
Template Set | Pistachio/Bkms_metabolic/Pistachio_ringbreaker/Reaxys/Reaxys_biocatalysis |
Top-N result to add to graph | 6 |
Feasible Synthetic Routes
Q1: What is the structural characterization of trilobatin 2″-acetate?
A1: Trilobatin 2″-acetate is a novel dihydrochalcone-glucoside. While its molecular formula and weight are not explicitly provided in the abstracts, its structure was elucidated using spectroscopic methods. These methods include one- and two-dimensional nuclear magnetic resonance spectroscopy (NMR) techniques like COSY, HMQC, and HMBC []. These techniques help determine the connectivity between atoms and provide crucial information about the compound's structure.
Disclaimer and Information on In-Vitro Research Products
Please be aware that all articles and product information presented on BenchChem are intended solely for informational purposes. The products available for purchase on BenchChem are specifically designed for in-vitro studies, which are conducted outside of living organisms. In-vitro studies, derived from the Latin term "in glass," involve experiments performed in controlled laboratory settings using cells or tissues. It is important to note that these products are not categorized as medicines or drugs, and they have not received approval from the FDA for the prevention, treatment, or cure of any medical condition, ailment, or disease. We must emphasize that any form of bodily introduction of these products into humans or animals is strictly prohibited by law. It is essential to adhere to these guidelines to ensure compliance with legal and ethical standards in research and experimentation.