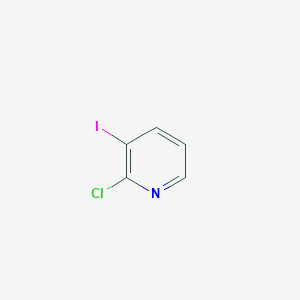
2-Chloro-3-iodopyridine
Overview
Description
2-Chloro-3-iodopyridine is an organic compound with the molecular formula C5H3ClIN. It is a halogenated pyridine derivative, characterized by the presence of both chlorine and iodine atoms on the pyridine ring. This compound is of significant interest in organic synthesis due to its unique reactivity and versatility as a building block in the preparation of various pharmaceuticals, agrochemicals, and advanced materials .
Mechanism of Action
Target of Action
2-Chloro-3-iodopyridine is a compound that has garnered significant attention in the field of chemistry, particularly in pharmaceutical research and development . Its unique structure, characterized by the presence of both chlorine and iodine atoms on the pyridine ring, renders it a valuable building block in organic synthesis . The compound’s primary targets are the molecules it interacts with in chemical reactions, primarily due to the presence of both a reactive amine group and halogen atoms .
Mode of Action
The mechanism of action of this compound in chemical reactions is a cornerstone of its utility in various fields . This compound plays a critical role primarily due to the presence of both a reactive amine group and halogen atoms, which offer multiple pathways for chemical interactions and transformations . At its core, this compound acts as a versatile nucleophile and electrophile, thanks to its amine group and halogen atoms, respectively .
Biochemical Pathways
The compound exhibits typical reactivity patterns of halogenated aromatics and amines . Its reactivity is further influenced by the presence of both electron-withdrawing and electron-donating groups, leading to a unique set of reactions and pathways that it can undergo . This reactivity profile is crucial in its applications, especially in organic synthesis, where it acts as an intermediate or a reactant .
Pharmacokinetics
The physical properties of this compound are noteworthy. It typically appears as a crystalline solid under standard conditions . The polar nature of the compound, owing to its halogen atoms and amine group, influences its solubility in various solvents, an important consideration in chemical synthesis and applications . .
Result of Action
The molecular and cellular effects of this compound’s action are primarily seen in its role as an intermediate in the synthesis of a wide range of pharmaceuticals, agrochemicals, and advanced materials . Its unique electronic properties affect its reactivity and interaction with other compounds, leading to the creation of new molecules with potential therapeutic significance .
Action Environment
The action, efficacy, and stability of this compound can be influenced by various environmental factors. For instance, the compound’s reactivity can be affected by the pH, temperature, and solvent used in the reaction .
Preparation Methods
Synthetic Routes and Reaction Conditions: 2-Chloro-3-iodopyridine can be synthesized through several methods. One common approach involves the halogenation of pyridine derivatives. For instance, 2-chloropyridine can be iodinated at the third position using iodine and a suitable catalyst such as iodotrimethylsilane . The reaction typically occurs under mild conditions, ensuring high yields and purity of the product.
Industrial Production Methods: In industrial settings, the production of this compound often involves large-scale halogenation processes. These processes are optimized for efficiency and cost-effectiveness, utilizing continuous flow reactors and advanced catalytic systems to achieve high throughput and consistent product quality .
Chemical Reactions Analysis
Types of Reactions: 2-Chloro-3-iodopyridine undergoes various chemical reactions, including:
Substitution Reactions: The chlorine and iodine atoms can be substituted with other functional groups, making it a versatile intermediate in organic synthesis.
Coupling Reactions: It participates in coupling reactions such as Suzuki, Stille, and Negishi couplings, forming carbon-carbon bonds with other aromatic or aliphatic compounds.
Common Reagents and Conditions:
Substitution Reactions: Reagents like sodium iodide or potassium fluoride are commonly used under mild conditions.
Coupling Reactions: Palladium or nickel catalysts are often employed in the presence of bases like potassium carbonate or cesium carbonate.
Major Products: The major products formed from these reactions include various substituted pyridines, which are valuable intermediates in the synthesis of pharmaceuticals and agrochemicals .
Scientific Research Applications
2-Chloro-3-iodopyridine has a wide range of applications in scientific research:
Comparison with Similar Compounds
2-Iodopyridine: Similar to 2-Chloro-3-iodopyridine but lacks the chlorine atom, making it less versatile in certain reactions.
3-Iodopyridine: Iodine is positioned at the third carbon, altering its reactivity and applications compared to this compound.
2-Chloropyridine: Contains only a chlorine atom, limiting its use in reactions that require iodine.
Uniqueness: this compound’s uniqueness lies in its dual halogenation, which provides a balance of reactivity and stability, making it a valuable intermediate in various synthetic pathways .
Properties
IUPAC Name |
2-chloro-3-iodopyridine | |
---|---|---|
Source | PubChem | |
URL | https://pubchem.ncbi.nlm.nih.gov | |
Description | Data deposited in or computed by PubChem | |
InChI |
InChI=1S/C5H3ClIN/c6-5-4(7)2-1-3-8-5/h1-3H | |
Source | PubChem | |
URL | https://pubchem.ncbi.nlm.nih.gov | |
Description | Data deposited in or computed by PubChem | |
InChI Key |
OHWSWGXNZDSHLM-UHFFFAOYSA-N | |
Source | PubChem | |
URL | https://pubchem.ncbi.nlm.nih.gov | |
Description | Data deposited in or computed by PubChem | |
Canonical SMILES |
C1=CC(=C(N=C1)Cl)I | |
Source | PubChem | |
URL | https://pubchem.ncbi.nlm.nih.gov | |
Description | Data deposited in or computed by PubChem | |
Molecular Formula |
C5H3ClIN | |
Source | PubChem | |
URL | https://pubchem.ncbi.nlm.nih.gov | |
Description | Data deposited in or computed by PubChem | |
DSSTOX Substance ID |
DTXSID40370916 | |
Record name | 2-chloro-3-iodopyridine | |
Source | EPA DSSTox | |
URL | https://comptox.epa.gov/dashboard/DTXSID40370916 | |
Description | DSSTox provides a high quality public chemistry resource for supporting improved predictive toxicology. | |
Molecular Weight |
239.44 g/mol | |
Source | PubChem | |
URL | https://pubchem.ncbi.nlm.nih.gov | |
Description | Data deposited in or computed by PubChem | |
CAS No. |
78607-36-0 | |
Record name | 2-chloro-3-iodopyridine | |
Source | EPA DSSTox | |
URL | https://comptox.epa.gov/dashboard/DTXSID40370916 | |
Description | DSSTox provides a high quality public chemistry resource for supporting improved predictive toxicology. | |
Record name | 2-Chloro-3-iodopyridine | |
Source | European Chemicals Agency (ECHA) | |
URL | https://echa.europa.eu/information-on-chemicals | |
Description | The European Chemicals Agency (ECHA) is an agency of the European Union which is the driving force among regulatory authorities in implementing the EU's groundbreaking chemicals legislation for the benefit of human health and the environment as well as for innovation and competitiveness. | |
Explanation | Use of the information, documents and data from the ECHA website is subject to the terms and conditions of this Legal Notice, and subject to other binding limitations provided for under applicable law, the information, documents and data made available on the ECHA website may be reproduced, distributed and/or used, totally or in part, for non-commercial purposes provided that ECHA is acknowledged as the source: "Source: European Chemicals Agency, http://echa.europa.eu/". Such acknowledgement must be included in each copy of the material. ECHA permits and encourages organisations and individuals to create links to the ECHA website under the following cumulative conditions: Links can only be made to webpages that provide a link to the Legal Notice page. | |
Retrosynthesis Analysis
AI-Powered Synthesis Planning: Our tool employs the Template_relevance Pistachio, Template_relevance Bkms_metabolic, Template_relevance Pistachio_ringbreaker, Template_relevance Reaxys, Template_relevance Reaxys_biocatalysis model, leveraging a vast database of chemical reactions to predict feasible synthetic routes.
One-Step Synthesis Focus: Specifically designed for one-step synthesis, it provides concise and direct routes for your target compounds, streamlining the synthesis process.
Accurate Predictions: Utilizing the extensive PISTACHIO, BKMS_METABOLIC, PISTACHIO_RINGBREAKER, REAXYS, REAXYS_BIOCATALYSIS database, our tool offers high-accuracy predictions, reflecting the latest in chemical research and data.
Strategy Settings
Precursor scoring | Relevance Heuristic |
---|---|
Min. plausibility | 0.01 |
Model | Template_relevance |
Template Set | Pistachio/Bkms_metabolic/Pistachio_ringbreaker/Reaxys/Reaxys_biocatalysis |
Top-N result to add to graph | 6 |
Feasible Synthetic Routes
Q1: What makes 2-chloro-3-iodopyridine a valuable compound in organic synthesis?
A1: this compound serves as a versatile building block for synthesizing various complex molecules, particularly heterocycles. Its value stems from the distinct reactivity of the chlorine and iodine substituents. These halogens can participate in diverse reactions, including palladium-catalyzed cross-coupling reactions like the Suzuki, Stille, and Buchwald-Hartwig reactions. [, , ] This allows for the selective introduction of various substituents at the 2- and 3-positions of the pyridine ring. [, , ]
Q2: What are some examples of heterocyclic compounds synthesized using this compound?
A2: Researchers have successfully employed this compound in synthesizing a range of heterocyclic compounds, including:
- Benzo[4,5]furopyridines: All four possible isomers of this tricyclic heterocycle have been synthesized using palladium-mediated reactions starting from this compound and related derivatives. []
- Dipyrido[1,2-a:3',2'-d]imidazoles: These compounds, along with their benzo- and aza-analogues, have been synthesized via a tandem double palladium-catalyzed amination of this compound with aminoazines and -diazines. []
- Imidazo[4,5-b]pyridin-2-ones: These compounds, including both disubstituted and pseudosymmetrically disubstituted derivatives, can be synthesized through a series of palladium-catalyzed aminations and cyclization reactions starting from this compound. []
- Fused polyaromatic alkaloids: Researchers have utilized the reactivity of the iodine substituent in this compound for heteroring cross-coupling reactions, leading to the synthesis of compounds like perlolidine, δ-carbolines, and 2,10-diazaphenanthrenes. []
Q3: Can you explain the “halogen-dance” phenomenon observed with this compound?
A3: "Halogen-dance" refers to the migration of a halogen atom on an aromatic ring. In the case of this compound, treatment with lithium diisopropylamide (LDA) at low temperatures leads to ortho-lithiation directed by the iodine atom. [] This is followed by a rapid ortho-migration of the iodine, resulting in a more stable iodolithiopyridine species. [] This phenomenon provides a valuable tool for regioselective functionalization of the pyridine ring, as the resulting lithiated species can react with various electrophiles. []
Q4: How is this compound typically synthesized?
A4: The synthesis of this compound involves a two-step process starting from 2-chloro-3-pyridinamine. [] The first step is diazotization, converting the amine group to a diazonium salt. [] Subsequently, the diazonium salt undergoes iodination to yield this compound with a yield of approximately 80%. [] The structure of the synthesized compound is confirmed using spectroscopic techniques like 1H NMR and IR. []
Disclaimer and Information on In-Vitro Research Products
Please be aware that all articles and product information presented on BenchChem are intended solely for informational purposes. The products available for purchase on BenchChem are specifically designed for in-vitro studies, which are conducted outside of living organisms. In-vitro studies, derived from the Latin term "in glass," involve experiments performed in controlled laboratory settings using cells or tissues. It is important to note that these products are not categorized as medicines or drugs, and they have not received approval from the FDA for the prevention, treatment, or cure of any medical condition, ailment, or disease. We must emphasize that any form of bodily introduction of these products into humans or animals is strictly prohibited by law. It is essential to adhere to these guidelines to ensure compliance with legal and ethical standards in research and experimentation.