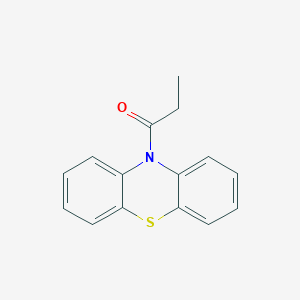
10-Propionylphenothiazine
Overview
Description
10-Propionylphenothiazine is a derivative of phenothiazine, characterized by the presence of a propionyl group at the tenth position of the phenothiazine ring.
Mechanism of Action
Target of Action
10-Propionylphenothiazine primarily targets Histamine H1 receptor , Muscarinic acetylcholine receptor , Alpha-1A adrenergic receptor , Dopamine D2 receptor , 5-hydroxytryptamine receptor 2A , and 5-hydroxytryptamine receptor 2C . These receptors play crucial roles in various physiological processes, including neurotransmission, immune response, and regulation of mood and cognition.
Mode of Action
This compound acts as an antagonist of the aforementioned receptors . By binding to these receptors, it inhibits their normal function, leading to a variety of physiological changes. For instance, its antihistamine effect is primarily responsible for its sedative properties .
Biochemical Pathways
This compound affects several biochemical pathways. It is known to inhibit enzymes in various intracellular biochemical pathways, including those activated by calmodulin . This inhibition can lead to a decrease in cell proliferation and inhibition of the P-glycoprotein transport function .
Pharmacokinetics
It is soluble in acetone, chloroform, dmso, and ethyl acetate , suggesting that it may have good bioavailability
Result of Action
The molecular and cellular effects of this compound’s action are diverse, given its wide range of targets. Its antagonistic action on various receptors can lead to changes in neurotransmission, immune response, and other physiological processes . It has been suggested as a potential cholinesterase inhibitor , which could have implications for the treatment of conditions like Alzheimer’s disease.
Action Environment
The action, efficacy, and stability of this compound can be influenced by various environmental factors. For instance, its solubility in various solvents suggests that it may be more effective in certain physiological environments . Additionally, factors such as pH, temperature, and the presence of other molecules could potentially affect its stability and action.
Biochemical Analysis
Biochemical Properties
The biochemical properties of 10-Propionylphenothiazine are not fully understood. It is known that phenothiazines, the parent compound, interact with various enzymes and proteins. For instance, they have been found to inhibit calmodulin, a protein that plays a key role in cellular processes
Cellular Effects
Phenothiazines have been shown to have pleiotropic effects on bacterial cells, including reducing cellular metabolic, transcriptional, and translational activities
Molecular Mechanism
Phenothiazines, the parent compound, are known to exert their effects through various mechanisms, including binding interactions with biomolecules, enzyme inhibition or activation, and changes in gene expression . It is plausible that this compound may exert its effects through similar mechanisms.
Metabolic Pathways
Phenothiazines, the parent compound, are known to be involved in various metabolic pathways
Preparation Methods
Synthetic Routes and Reaction Conditions: The synthesis of 10-Propionylphenothiazine typically involves the acylation of phenothiazine with propionyl chloride in the presence of a Lewis acid catalyst such as aluminum chloride. The reaction is carried out under anhydrous conditions to prevent hydrolysis of the acyl chloride. The general reaction scheme is as follows:
Phenothiazine+Propionyl ChlorideAlCl3this compound+HCl
Industrial Production Methods: On an industrial scale, the production of this compound follows similar synthetic routes but with optimized reaction conditions to ensure higher yields and purity. The process involves continuous monitoring of reaction parameters such as temperature, pressure, and reactant concentrations. The product is then purified using techniques like recrystallization or chromatography .
Chemical Reactions Analysis
Types of Reactions: 10-Propionylphenothiazine undergoes various chemical reactions, including:
Oxidation: The compound can be oxidized to form sulfoxides or sulfones.
Reduction: Reduction reactions can convert the carbonyl group to an alcohol.
Substitution: Electrophilic substitution reactions can occur at the aromatic ring, leading to the formation of various derivatives.
Common Reagents and Conditions:
Oxidation: Reagents like hydrogen peroxide or peracids are commonly used.
Reduction: Reducing agents such as lithium aluminum hydride or sodium borohydride are employed.
Substitution: Halogenating agents or nitrating agents are used under controlled conditions.
Major Products Formed:
Oxidation: Sulfoxides and sulfones.
Reduction: Alcohol derivatives.
Substitution: Halogenated or nitrated phenothiazine derivatives.
Scientific Research Applications
10-Propionylphenothiazine has found applications in various scientific research fields:
Chemistry: Used as a precursor for synthesizing other phenothiazine derivatives.
Biology: Investigated for its potential as a cholinesterase inhibitor, which could have implications in treating neurodegenerative diseases.
Medicine: Explored for its antipsychotic and anti-inflammatory properties.
Industry: Utilized in the development of dyes and pigments due to its stable chemical structure
Comparison with Similar Compounds
Phenothiazine: The parent compound, known for its antipsychotic properties.
Chlorpromazine: A widely used antipsychotic drug.
Trifluoperazine: Another phenothiazine derivative with antipsychotic effects.
Comparison: 10-Propionylphenothiazine is unique due to the presence of the propionyl group, which modifies its pharmacological profile. Compared to phenothiazine, it has enhanced cholinesterase inhibitory activity. Unlike chlorpromazine and trifluoperazine, this compound is less commonly used in clinical settings but holds potential for future therapeutic applications .
Properties
IUPAC Name |
1-phenothiazin-10-ylpropan-1-one | |
---|---|---|
Source | PubChem | |
URL | https://pubchem.ncbi.nlm.nih.gov | |
Description | Data deposited in or computed by PubChem | |
InChI |
InChI=1S/C15H13NOS/c1-2-15(17)16-11-7-3-5-9-13(11)18-14-10-6-4-8-12(14)16/h3-10H,2H2,1H3 | |
Source | PubChem | |
URL | https://pubchem.ncbi.nlm.nih.gov | |
Description | Data deposited in or computed by PubChem | |
InChI Key |
NCDUBBOHHQXHIQ-UHFFFAOYSA-N | |
Source | PubChem | |
URL | https://pubchem.ncbi.nlm.nih.gov | |
Description | Data deposited in or computed by PubChem | |
Canonical SMILES |
CCC(=O)N1C2=CC=CC=C2SC3=CC=CC=C31 | |
Source | PubChem | |
URL | https://pubchem.ncbi.nlm.nih.gov | |
Description | Data deposited in or computed by PubChem | |
Molecular Formula |
C15H13NOS | |
Source | PubChem | |
URL | https://pubchem.ncbi.nlm.nih.gov | |
Description | Data deposited in or computed by PubChem | |
DSSTOX Substance ID |
DTXSID90216416 | |
Record name | 10-Propionylphenothiazine | |
Source | EPA DSSTox | |
URL | https://comptox.epa.gov/dashboard/DTXSID90216416 | |
Description | DSSTox provides a high quality public chemistry resource for supporting improved predictive toxicology. | |
Molecular Weight |
255.3 g/mol | |
Source | PubChem | |
URL | https://pubchem.ncbi.nlm.nih.gov | |
Description | Data deposited in or computed by PubChem | |
CAS No. |
6622-75-9 | |
Record name | 1-(10H-Phenothiazin-10-yl)-1-propanone | |
Source | CAS Common Chemistry | |
URL | https://commonchemistry.cas.org/detail?cas_rn=6622-75-9 | |
Description | CAS Common Chemistry is an open community resource for accessing chemical information. Nearly 500,000 chemical substances from CAS REGISTRY cover areas of community interest, including common and frequently regulated chemicals, and those relevant to high school and undergraduate chemistry classes. This chemical information, curated by our expert scientists, is provided in alignment with our mission as a division of the American Chemical Society. | |
Explanation | The data from CAS Common Chemistry is provided under a CC-BY-NC 4.0 license, unless otherwise stated. | |
Record name | 10-Propionylphenothiazine | |
Source | ChemIDplus | |
URL | https://pubchem.ncbi.nlm.nih.gov/substance/?source=chemidplus&sourceid=0006622759 | |
Description | ChemIDplus is a free, web search system that provides access to the structure and nomenclature authority files used for the identification of chemical substances cited in National Library of Medicine (NLM) databases, including the TOXNET system. | |
Record name | 6622-75-9 | |
Source | DTP/NCI | |
URL | https://dtp.cancer.gov/dtpstandard/servlet/dwindex?searchtype=NSC&outputformat=html&searchlist=55093 | |
Description | The NCI Development Therapeutics Program (DTP) provides services and resources to the academic and private-sector research communities worldwide to facilitate the discovery and development of new cancer therapeutic agents. | |
Explanation | Unless otherwise indicated, all text within NCI products is free of copyright and may be reused without our permission. Credit the National Cancer Institute as the source. | |
Record name | 10-Propionylphenothiazine | |
Source | EPA DSSTox | |
URL | https://comptox.epa.gov/dashboard/DTXSID90216416 | |
Description | DSSTox provides a high quality public chemistry resource for supporting improved predictive toxicology. | |
Record name | 10-propionylphenothiazine | |
Source | European Chemicals Agency (ECHA) | |
URL | https://echa.europa.eu/substance-information/-/substanceinfo/100.026.886 | |
Description | The European Chemicals Agency (ECHA) is an agency of the European Union which is the driving force among regulatory authorities in implementing the EU's groundbreaking chemicals legislation for the benefit of human health and the environment as well as for innovation and competitiveness. | |
Explanation | Use of the information, documents and data from the ECHA website is subject to the terms and conditions of this Legal Notice, and subject to other binding limitations provided for under applicable law, the information, documents and data made available on the ECHA website may be reproduced, distributed and/or used, totally or in part, for non-commercial purposes provided that ECHA is acknowledged as the source: "Source: European Chemicals Agency, http://echa.europa.eu/". Such acknowledgement must be included in each copy of the material. ECHA permits and encourages organisations and individuals to create links to the ECHA website under the following cumulative conditions: Links can only be made to webpages that provide a link to the Legal Notice page. | |
Retrosynthesis Analysis
AI-Powered Synthesis Planning: Our tool employs the Template_relevance Pistachio, Template_relevance Bkms_metabolic, Template_relevance Pistachio_ringbreaker, Template_relevance Reaxys, Template_relevance Reaxys_biocatalysis model, leveraging a vast database of chemical reactions to predict feasible synthetic routes.
One-Step Synthesis Focus: Specifically designed for one-step synthesis, it provides concise and direct routes for your target compounds, streamlining the synthesis process.
Accurate Predictions: Utilizing the extensive PISTACHIO, BKMS_METABOLIC, PISTACHIO_RINGBREAKER, REAXYS, REAXYS_BIOCATALYSIS database, our tool offers high-accuracy predictions, reflecting the latest in chemical research and data.
Strategy Settings
Precursor scoring | Relevance Heuristic |
---|---|
Min. plausibility | 0.01 |
Model | Template_relevance |
Template Set | Pistachio/Bkms_metabolic/Pistachio_ringbreaker/Reaxys/Reaxys_biocatalysis |
Top-N result to add to graph | 6 |
Feasible Synthetic Routes
Disclaimer and Information on In-Vitro Research Products
Please be aware that all articles and product information presented on BenchChem are intended solely for informational purposes. The products available for purchase on BenchChem are specifically designed for in-vitro studies, which are conducted outside of living organisms. In-vitro studies, derived from the Latin term "in glass," involve experiments performed in controlled laboratory settings using cells or tissues. It is important to note that these products are not categorized as medicines or drugs, and they have not received approval from the FDA for the prevention, treatment, or cure of any medical condition, ailment, or disease. We must emphasize that any form of bodily introduction of these products into humans or animals is strictly prohibited by law. It is essential to adhere to these guidelines to ensure compliance with legal and ethical standards in research and experimentation.