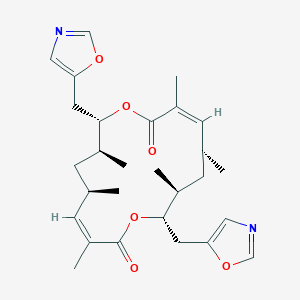
3,5,7,11,13,15-hexamethyl-8,16-bis(1,3-oxazol-5-ylmethyl)-1,9-dioxacyclohexadeca-3,11-diene-2,10-dione
- Click on QUICK INQUIRY to receive a quote from our team of experts.
- With the quality product at a COMPETITIVE price, you can focus more on your research.
Overview
Description
Conglobatin is a unique C2-symmetrical macrodiolide derived from the bacterium Streptomyces conglobatus. It is known for its promising antitumor activity and has been a subject of interest in the field of natural product chemistry due to its complex structure and biological properties .
Mechanism of Action
Conglobatin is a unique C2-symmetrical macrodiolide derived from the bacterium Streptomyces conglobatus, exhibiting promising antitumor activity . This article will delve into the mechanism of action of Conglobatin, covering its primary targets, mode of action, affected biochemical pathways, pharmacokinetics, molecular and cellular effects, and the influence of environmental factors on its action.
Target of Action
Conglobatin primarily targets the Heat Shock Protein 90 (Hsp90) and its co-chaperone Cell Division Cycle 37 (Cdc37) . Hsp90 is a chaperone protein that plays a crucial role in the folding, stability, and function of many proteins, including kinases, hormone receptors, and transcription factors . Cdc37 is a co-chaperone that assists Hsp90 in the folding of protein kinases .
Mode of Action
Conglobatin interacts with its targets by binding to the N-terminal domain of Hsp90, disrupting the Hsp90-Cdc37 complex formation . This disruption inhibits the interaction between Hsp90 and Cdc37, which is crucial for the stability and function of many client proteins . By inhibiting this interaction, Conglobatin can disrupt the normal functioning of these proteins, leading to potential antitumor effects .
Biochemical Pathways
The disruption of the Hsp90-Cdc37 interaction by Conglobatin affects the stability and function of many client proteins, including kinases, hormone receptors, and transcription factors . These proteins are involved in various biochemical pathways, including cell growth, differentiation, and apoptosis . By disrupting these pathways, Conglobatin can exert its antitumor effects .
Pharmacokinetics
It is known that conglobatin is an orally active compound , suggesting that it has good bioavailability
Result of Action
Conglobatin’s disruption of the Hsp90-Cdc37 interaction leads to the destabilization and dysfunction of many client proteins . This can result in the inhibition of cell growth and induction of apoptosis, contributing to its antitumor effects . In addition, Conglobatin has been shown to enhance the cytotoxicity of chemotherapeutic agents in cancer cells overexpressing ABCB1 or ABCG2, suggesting a potential role in reversing multidrug resistance .
Action Environment
It is known that the efficacy and stability of many drugs can be influenced by factors such as ph, temperature, and the presence of other substances
Biochemical Analysis
Biochemical Properties
Conglobatin interacts with various biomolecules in biochemical reactions. It diverges from its analogues through differing patterns of methylation on the macrodiolide skeleton . The altered methyl positions suggest a deviation from the published biosynthetic pathway, which proposed three successive methylmalonyl-CoA extender unit additions to the Conglobatin monomer .
Cellular Effects
Conglobatin exhibits potent cytotoxic activity selectively against certain cell lines. For instance, Conglobatins B1, C1, and C2 have shown more potent cytotoxic activity selectively against the NS-1 myeloma cell line compared with Conglobatin .
Molecular Mechanism
The molecular mechanism of Conglobatin involves the cyclase/thioesterase acting iteratively, ligating two monomers head-to-tail, then re-binding the dimer product and cyclizing it . This process is directed by the Conglobatin cluster in a heterologous host strain .
Metabolic Pathways
Conglobatin is involved in complex metabolic pathways. The biosynthesis of Conglobatin in Streptomyces conglobatus involves a modular polyketide synthase assembly line
Preparation Methods
Synthetic Routes and Reaction Conditions: Conglobatin is biosynthesized through a polyketide synthase (PKS) pathway. The biosynthetic gene cluster responsible for conglobatin production has been cloned and expressed in a heterologous host, which allows for the detailed study of its biosynthesis . The process involves the iterative action of a cyclase/thioesterase enzyme that ligates two monomers head-to-tail and then cyclizes the dimer product .
Industrial Production Methods: Industrial production of conglobatin is primarily achieved through fermentation of the Streptomyces conglobatus strain. Optimization of fermentation conditions, such as nutrient composition, pH, and temperature, is crucial for maximizing yield .
Chemical Reactions Analysis
Types of Reactions: Conglobatin undergoes various chemical reactions, including:
Reduction: Involves the removal of oxygen atoms or the addition of hydrogen atoms.
Substitution: Involves the replacement of one functional group with another.
Common Reagents and Conditions:
Oxidation: Common reagents include potassium permanganate and chromium trioxide.
Reduction: Common reagents include lithium aluminum hydride and sodium borohydride.
Substitution: Common reagents include halogens and nucleophiles.
Major Products: The major products formed from these reactions depend on the specific conditions and reagents used. For example, oxidation may yield hydroxylated derivatives, while reduction may yield deoxygenated derivatives .
Scientific Research Applications
Conglobatin has a wide range of scientific research applications, including:
Chemistry: Used as a model compound for studying polyketide biosynthesis and macrodiolide formation.
Biology: Studied for its cytotoxic activity against various cancer cell lines, including myeloma cells.
Industry: Potential applications in the development of new pharmaceuticals and agrochemicals.
Comparison with Similar Compounds
Conglobatin is unique due to its C2-symmetrical macrodiolide structure. Similar compounds include:
Elaiophylins: Another family of 16-membered diene-conjugated lactones with symmetrical lactone arrangements.
Oxazolomycin: An oxazole triene antibiotic with potent cytotoxic and antibacterial activity.
Conglobatin stands out due to its specific inhibition of the Hsp90/Cdc37 protein interface, which is not a common mechanism among similar compounds .
Properties
CAS No. |
72263-05-9 |
---|---|
Molecular Formula |
C28H38N2O6 |
Molecular Weight |
498.6 g/mol |
IUPAC Name |
(3Z,5R,7S,8S,11Z,13R,15S,16S)-3,5,7,11,13,15-hexamethyl-8,16-bis(1,3-oxazol-5-ylmethyl)-1,9-dioxacyclohexadeca-3,11-diene-2,10-dione |
InChI |
InChI=1S/C28H38N2O6/c1-17-7-19(3)25(11-23-13-29-15-33-23)35-28(32)22(6)10-18(2)8-20(4)26(12-24-14-30-16-34-24)36-27(31)21(5)9-17/h9-10,13-20,25-26H,7-8,11-12H2,1-6H3/b21-9-,22-10-/t17-,18-,19+,20+,25+,26+/m1/s1 |
InChI Key |
LAJRJVDLKYGLOO-NLISZJEWSA-N |
SMILES |
CC1CC(C(OC(=O)C(=CC(CC(C(OC(=O)C(=C1)C)CC2=CN=CO2)C)C)C)CC3=CN=CO3)C |
Isomeric SMILES |
C[C@H]1/C=C(\C(=O)O[C@H]([C@H](C[C@H](/C=C(\C(=O)O[C@H]([C@H](C1)C)CC2=CN=CO2)/C)C)C)CC3=CN=CO3)/C |
Canonical SMILES |
CC1CC(C(OC(=O)C(=CC(CC(C(OC(=O)C(=C1)C)CC2=CN=CO2)C)C)C)CC3=CN=CO3)C |
Synonyms |
(3E,5R,11E)-3,5,7S,11,13R,15S-hexamethyl-8S,16S-bis(5-oxazolylmethyl)-1,9-dioxacyclohexadeca-3,11-diene-2,10-dione |
Origin of Product |
United States |
Retrosynthesis Analysis
AI-Powered Synthesis Planning: Our tool employs the Template_relevance Pistachio, Template_relevance Bkms_metabolic, Template_relevance Pistachio_ringbreaker, Template_relevance Reaxys, Template_relevance Reaxys_biocatalysis model, leveraging a vast database of chemical reactions to predict feasible synthetic routes.
One-Step Synthesis Focus: Specifically designed for one-step synthesis, it provides concise and direct routes for your target compounds, streamlining the synthesis process.
Accurate Predictions: Utilizing the extensive PISTACHIO, BKMS_METABOLIC, PISTACHIO_RINGBREAKER, REAXYS, REAXYS_BIOCATALYSIS database, our tool offers high-accuracy predictions, reflecting the latest in chemical research and data.
Strategy Settings
Precursor scoring | Relevance Heuristic |
---|---|
Min. plausibility | 0.01 |
Model | Template_relevance |
Template Set | Pistachio/Bkms_metabolic/Pistachio_ringbreaker/Reaxys/Reaxys_biocatalysis |
Top-N result to add to graph | 6 |
Feasible Synthetic Routes
Disclaimer and Information on In-Vitro Research Products
Please be aware that all articles and product information presented on BenchChem are intended solely for informational purposes. The products available for purchase on BenchChem are specifically designed for in-vitro studies, which are conducted outside of living organisms. In-vitro studies, derived from the Latin term "in glass," involve experiments performed in controlled laboratory settings using cells or tissues. It is important to note that these products are not categorized as medicines or drugs, and they have not received approval from the FDA for the prevention, treatment, or cure of any medical condition, ailment, or disease. We must emphasize that any form of bodily introduction of these products into humans or animals is strictly prohibited by law. It is essential to adhere to these guidelines to ensure compliance with legal and ethical standards in research and experimentation.