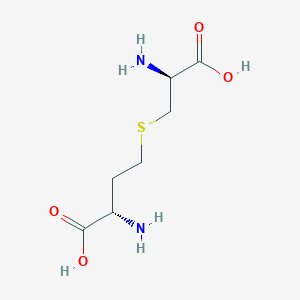
D-allocystathionine
Overview
Description
D-Allocystathionine is a sulfur-containing amino acid derivative that plays a significant role in the sulfur metabolism pathway. It is a product of the enzyme cystathionine synthetase, which converts homocysteine into allocystathionine. This compound is also a substrate for the enzyme cystathionine beta-lyase .
Preparation Methods
Synthetic Routes and Reaction Conditions: D-Allocystathionine can be synthesized through enzymatic reactions involving cystathionine synthetase and cystathionine beta-lyase. The synthesis typically involves the conversion of homocysteine to allocystathionine in the presence of these enzymes .
Industrial Production Methods: This method ensures high yield and purity of the final product .
Chemical Reactions Analysis
Types of Reactions: D-Allocystathionine undergoes various chemical reactions, including:
Oxidation: This reaction involves the addition of oxygen or the removal of hydrogen, leading to the formation of sulfoxides or sulfones.
Reduction: This reaction involves the gain of electrons or hydrogen, resulting in the formation of thiols or sulfides.
Common Reagents and Conditions:
Oxidation: Common reagents include hydrogen peroxide and peracids.
Reduction: Common reagents include sodium borohydride and lithium aluminum hydride.
Substitution: Common reagents include alkyl halides and acyl chlorides.
Major Products Formed:
Oxidation: Sulfoxides and sulfones.
Reduction: Thiols and sulfides.
Substitution: Various substituted derivatives depending on the reagents used.
Scientific Research Applications
D-Allocystathionine has several applications in scientific research, including:
Chemistry: Used as a precursor in the synthesis of other sulfur-containing compounds.
Biology: Studied for its role in sulfur metabolism and its impact on cellular processes.
Medicine: Investigated for its potential therapeutic effects in conditions related to sulfur metabolism disorders.
Industry: Utilized in the production of fine chemicals and as a biocatalyst in various industrial processes
Mechanism of Action
D-Allocystathionine exerts its effects through its involvement in the sulfur metabolism pathway. It is converted from homocysteine by cystathionine synthetase and further metabolized by cystathionine beta-lyase. These enzymatic reactions are crucial for maintaining sulfur balance in the body and for the synthesis of other essential sulfur-containing compounds .
Comparison with Similar Compounds
L-Cystathionine: Another sulfur-containing amino acid derivative involved in the same metabolic pathway.
L-Allocystathionine: The L-isomer of D-Allocystathionine, with similar chemical properties but different biological activity.
L-Homocysteine: A precursor in the synthesis of both this compound and L-Cystathionine.
Uniqueness: this compound is unique due to its specific stereochemistry, which influences its interaction with enzymes and its role in metabolic pathways. Its distinct configuration allows it to participate in specific biochemical reactions that are not possible with its isomers .
Properties
IUPAC Name |
(2S)-2-amino-4-[(2S)-2-amino-2-carboxyethyl]sulfanylbutanoic acid | |
---|---|---|
Source | PubChem | |
URL | https://pubchem.ncbi.nlm.nih.gov | |
Description | Data deposited in or computed by PubChem | |
InChI |
InChI=1S/C7H14N2O4S/c8-4(6(10)11)1-2-14-3-5(9)7(12)13/h4-5H,1-3,8-9H2,(H,10,11)(H,12,13)/t4-,5+/m0/s1 | |
Source | PubChem | |
URL | https://pubchem.ncbi.nlm.nih.gov | |
Description | Data deposited in or computed by PubChem | |
InChI Key |
ILRYLPWNYFXEMH-CRCLSJGQSA-N | |
Source | PubChem | |
URL | https://pubchem.ncbi.nlm.nih.gov | |
Description | Data deposited in or computed by PubChem | |
Canonical SMILES |
C(CSCC(C(=O)O)N)C(C(=O)O)N | |
Source | PubChem | |
URL | https://pubchem.ncbi.nlm.nih.gov | |
Description | Data deposited in or computed by PubChem | |
Isomeric SMILES |
C(CSC[C@H](C(=O)O)N)[C@@H](C(=O)O)N | |
Source | PubChem | |
URL | https://pubchem.ncbi.nlm.nih.gov | |
Description | Data deposited in or computed by PubChem | |
Molecular Formula |
C7H14N2O4S | |
Source | PubChem | |
URL | https://pubchem.ncbi.nlm.nih.gov | |
Description | Data deposited in or computed by PubChem | |
DSSTOX Substance ID |
DTXSID10331433 | |
Record name | D-allocystathionine | |
Source | EPA DSSTox | |
URL | https://comptox.epa.gov/dashboard/DTXSID10331433 | |
Description | DSSTox provides a high quality public chemistry resource for supporting improved predictive toxicology. | |
Molecular Weight |
222.26 g/mol | |
Source | PubChem | |
URL | https://pubchem.ncbi.nlm.nih.gov | |
Description | Data deposited in or computed by PubChem | |
CAS No. |
2998-83-6 | |
Record name | D-allocystathionine | |
Source | EPA DSSTox | |
URL | https://comptox.epa.gov/dashboard/DTXSID10331433 | |
Description | DSSTox provides a high quality public chemistry resource for supporting improved predictive toxicology. | |
Retrosynthesis Analysis
AI-Powered Synthesis Planning: Our tool employs the Template_relevance Pistachio, Template_relevance Bkms_metabolic, Template_relevance Pistachio_ringbreaker, Template_relevance Reaxys, Template_relevance Reaxys_biocatalysis model, leveraging a vast database of chemical reactions to predict feasible synthetic routes.
One-Step Synthesis Focus: Specifically designed for one-step synthesis, it provides concise and direct routes for your target compounds, streamlining the synthesis process.
Accurate Predictions: Utilizing the extensive PISTACHIO, BKMS_METABOLIC, PISTACHIO_RINGBREAKER, REAXYS, REAXYS_BIOCATALYSIS database, our tool offers high-accuracy predictions, reflecting the latest in chemical research and data.
Strategy Settings
Precursor scoring | Relevance Heuristic |
---|---|
Min. plausibility | 0.01 |
Model | Template_relevance |
Template Set | Pistachio/Bkms_metabolic/Pistachio_ringbreaker/Reaxys/Reaxys_biocatalysis |
Top-N result to add to graph | 6 |
Feasible Synthetic Routes
Q1: What is the biological significance of D-allocystathionine and its relationship to other sulfur-containing amino acids?
A1: this compound is one of the four stereoisomers of cystathionine, a key intermediate in the metabolic pathway converting methionine to cysteine. [] While L-cystathionine plays a direct role in this pathway, the ability of this compound to participate in sulfur metabolism is less clear. Research indicates that this compound can be synthesized [] and might have distinct biological activities compared to its isomers. [] Further investigations are needed to fully elucidate its metabolic fate and potential roles in biological systems.
Q2: Can this compound be synthesized enzymatically, and if so, what enzymes and substrates are involved?
A2: Yes, this compound can be enzymatically synthesized using cystathionine γ-lyase from Streptomyces phaeochromogenes. [] This enzyme exhibits broad substrate specificity, catalyzing not only the α,γ-elimination of L-cystathionine but also γ-replacement reactions with L-homoserine and various thiol compounds. Researchers successfully synthesized this compound by reacting L-homoserine with D-cysteine in the presence of cystathionine γ-lyase. [] This enzymatic approach offers a promising alternative to traditional chemical synthesis methods.
Q3: How does the stereochemistry of methionine derivatives, particularly D-methionine, affect their utilization by specific strains of bacteria?
A3: Studies using a methionine-requiring Escherichia coli mutant (strain 58-161) revealed intriguing differences in the utilization of D- and L-methionine. [] Although both isomers supported growth, the growth rate was significantly slower with D-methionine compared to L-methionine. [] This suggests that the bacterial enzymes involved in methionine metabolism exhibit stereoselectivity, preferentially recognizing and processing the L-enantiomer. Further research is necessary to pinpoint the specific enzymatic steps influenced by methionine stereochemistry and their broader implications in bacterial metabolism.
Q4: What are the potential future directions for research on this compound?
A4: While the provided research offers valuable insights into this compound, several open questions warrant further investigation:
Disclaimer and Information on In-Vitro Research Products
Please be aware that all articles and product information presented on BenchChem are intended solely for informational purposes. The products available for purchase on BenchChem are specifically designed for in-vitro studies, which are conducted outside of living organisms. In-vitro studies, derived from the Latin term "in glass," involve experiments performed in controlled laboratory settings using cells or tissues. It is important to note that these products are not categorized as medicines or drugs, and they have not received approval from the FDA for the prevention, treatment, or cure of any medical condition, ailment, or disease. We must emphasize that any form of bodily introduction of these products into humans or animals is strictly prohibited by law. It is essential to adhere to these guidelines to ensure compliance with legal and ethical standards in research and experimentation.