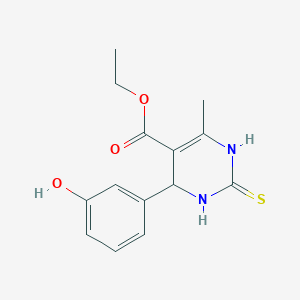
Monastrol
Overview
Description
Monastrol (ethyl 6-methyl-2-thioxo-1,2,3,4-tetrahydropyrimidine-5-carboxylate) is a dihydropyrimidinone (DHPM) compound synthesized via the Biginelli reaction from ethyl acetoacetate, thiourea, and 3-hydroxybenzaldehyde . Discovered through phenotypic cell-based screening, it was the first small-molecule allosteric inhibitor of the mitotic kinesin Eg5 (kinesin spindle protein, KSP), a motor protein critical for bipolar spindle assembly during mitosis . This compound binds to a hydrophobic pocket 12 Å away from the Eg5 catalytic site, inducing conformational changes that block ATPase activity and microtubule interactions, leading to mitotic arrest and monopolar spindle formation . Its IC50 for Eg5 inhibition is ~5.2 µM, and it exhibits species specificity due to sequence divergence in Eg5 homologs .
Preparation Methods
Conventional Biginelli Reaction and Microwave-Assisted Synthesis
Conventional Heating Methods
The traditional Biginelli reaction for Monastrol synthesis involves refluxing equimolar amounts of 3-hydroxybenzaldehyde, ethyl acetoacetate, and thiourea in ethanol under acidic conditions. This method typically requires prolonged reaction times (6–12 hours) and yields approximately 50–60% of the target compound . However, the formation of side products, such as dimeric byproducts, often necessitates additional purification steps, including recrystallization or column chromatography .
Microwave-Assisted Optimization
Microwave irradiation has revolutionized this compound synthesis by reducing reaction times and improving yields. Under sealed-vessel microwave conditions at 120°C, the Biginelli condensation is completed in 30 minutes , achieving a crude yield of 89% . Key advantages include:
-
Reduced side-product formation : Microwave heating minimizes thermal degradation, leading to purer crude products .
-
Scalability : Reactions can be performed in 0.5–2 mL microwave vials with magnetic stirring, ensuring reproducibility .
-
Energy efficiency : The rapid heating profile lowers overall energy consumption compared to conventional reflux .
Post-synthesis purification via precipitation/filtration (76% isolated yield) or column chromatography (86% yield) further enhances product purity .
Catalytic Systems for Enhanced Efficiency
Lewis Acid Catalysts
Lewis acids such as ErCl₃·6H₂O and FeCl₃ have been employed to accelerate the Biginelli reaction. Erbium trichloride hexahydrate, used in solvent-free conditions with a Q-tube reactor, achieves this compound in 85% yield within 1 hour . Similarly, FeCl₃ under solvent-free conditions improves yields to 78% compared to non-catalyzed reactions (52%) .
Table 1: Catalyst Performance in this compound Synthesis
Catalyst | Reaction Time | Yield (%) | Conditions | Reference |
---|---|---|---|---|
ErCl₃·6H₂O | 1 h | 85 | Solvent-free, Q-tube | |
FeCl₃ | 4 h | 78 | Solvent-free | |
CuCl₂ | 4 h | 72 | Solvent-free | |
HCl (Brønsted) | 4 h | 68 | Solvent-free |
Brønsted Acid Catalysts
Brønsted acids like HCl and CF₃COOH have also been explored. Trifluoroacetic acid (CF₃COOH) in ethanol increases reaction rates, achieving 70% yield in 3 hours . However, these methods often require neutralization steps, complicating workup procedures.
Green Catalytic Approaches
Eutectic mixtures and ionic liquids, such as choline chloride-urea, enable eco-friendly synthesis under microwave irradiation. These systems eliminate volatile organic solvents and achieve yields comparable to conventional methods (75–80%) .
Solvent-Free and Eco-Friendly Protocols
Solvent-Free Biginelli Reaction
Solvent-free conditions reduce environmental impact and simplify purification. For example, using ErCl₃·6H₂O in a Q-tube reactor under pressure yields this compound in 85% purity without additional solvents . This method aligns with green chemistry principles by minimizing waste generation.
Mechanochemical Synthesis
Ball-milling techniques, though less explored, offer a promising alternative. Preliminary studies show that mechanochemical activation can reduce reaction times to 45 minutes with yields of 70% .
Purification Techniques and Yield Optimization
Precipitation/Filtration vs. Column Chromatography
Microwave-synthesized this compound can be purified via two routes:
-
Precipitation/Filtration : Crude product is dissolved in hot ethanol and precipitated by cooling, yielding 76% pure this compound .
-
Column Chromatography : Silica gel chromatography with ethyl acetate/hexane eluents increases yield to 86% but adds time and cost .
Table 2: Purification Method Comparison
Method | Yield (%) | Purity | Time Required |
---|---|---|---|
Precipitation | 76 | High | 2 h |
Column Chromatography | 86 | Very High | 4–6 h |
Recrystallization
Ethanol-water recrystallization remains a standard method, yielding 70–75% pure this compound with melting points of 169–171°C .
Recent Advances and Structural Analogues
Synthesis of this compound Analogues
Modifying the this compound scaffold has led to derivatives with enhanced bioactivity. For instance, replacing the 3-hydroxyphenyl group with imidazole aldehydes yields analogues showing improved Eg5 inhibition (IC₅₀ = 2.1–4.3 μM) . These syntheses employ TMSCl/DMF/acetonitrile systems, achieving 36–44% yields after centrifugation .
High-Throughput Screening
Automated platforms utilizing 96-well plates and kinetic absorbance measurements (λ = 360 nm) enable rapid screening of this compound derivatives for kinesin inhibition .
Chemical Reactions Analysis
Types of Reactions: Monastrol undergoes various chemical reactions, including oxidation, reduction, and substitution. These reactions are critical for its biological activity and pharmacological effects.
Common Reagents and Conditions:Oxidation: this compound may be oxidized using specific reagents to modify its structure.
Reduction: Reduction reactions can alter this compound’s functional groups.
Substitution: Substituting specific atoms or groups in this compound can lead to derivatives with distinct properties.
Major Products: The major products formed from this compound reactions depend on the specific reaction conditions. Researchers have synthesized derivatives with varying substituents and functional groups to explore their effects.
Scientific Research Applications
Anticancer Applications
1. Cancer Cell Cycle Arrest
Monastrol has been extensively studied for its ability to induce mitotic arrest in various cancer cell lines, including breast (MCF-7), cervical (HeLa), and gastric (AGS) cancers. Research indicates that this compound-treated cells accumulate in mitosis with monoastral spindles, leading to apoptosis. The effectiveness varies among different cancer types, with AGS cells showing higher sensitivity compared to HT29 cells .
2. Development of Derivatives
Efforts to enhance the efficacy of this compound have led to the synthesis of various derivatives. These analogs have been evaluated for their cytotoxic effects against cancer cell lines, revealing improved potency compared to this compound itself. Structure-activity relationship (SAR) studies have guided the design of these compounds, focusing on modifications that enhance their inhibitory effects on Eg5 .
Non-Cancer Applications
1. Urease Inhibition
Recent studies have identified this compound as a potent urease inhibitor through structure-based virtual screening (SBVS). This discovery positions this compound derivatives as potential candidates for developing antiulcer drugs. The ability to inhibit urease may provide therapeutic benefits in conditions such as peptic ulcers and other gastrointestinal diseases .
2. Research Tool in Cell Biology
This compound serves as a valuable reagent for probing mitotic mechanisms beyond its therapeutic applications. Its ability to induce monoastral spindles allows researchers to study spindle assembly checkpoints and the dynamics of microtubule interactions during mitosis. This application has facilitated insights into chromosomal malorientation and the role of Eg5 in spindle maintenance .
Case Studies
Mechanism of Action
Monastrol’s mechanism involves inhibiting kinesin-5 (Eg5). By disrupting spindle bipolarity, it prevents proper cell division. Eg5 inhibition leads to collapsed spindles and cell cycle arrest .
Comparison with Similar Compounds
Monastrol serves as a structural and functional benchmark for Eg5 inhibitors. Below, its pharmacological and biochemical properties are compared to derivatives and structurally distinct Eg5 inhibitors.
Structural Derivatives of this compound
2.1.1. Thio- vs. Oxo-Derivatives
- Thio-derivatives (e.g., this compound): The thioxo group at position 2 enhances cytotoxicity. This compound (IC50: 5.2 µM) is more potent than its oxo-analogue (IC50: >25 µM) in growth inhibition assays, with sulfur critical for hydrophobic interactions in the Eg5 binding pocket .
- Oxo-derivatives: Exhibit cytostatic rather than cytotoxic effects, limiting their therapeutic utility .
2.1.2. Substituted Aromatic Moieties
- 3-Hydroxyphenyl Group: Essential for activity. Removal (e.g., derivative 19e) abolishes hydrogen bonding with Glu118, reducing Eg5 inhibition . Derivatives retaining this group (e.g., dimethylenastron) show improved potency .
- Nitrophenyl Substituents (LaSOM 65): A 3-nitrophenyl derivative with >70% antitumor activity in murine Sarcoma 180 models, surpassing this compound’s efficacy .
2.1.3. Fatty Acid Hybrids
- Palmitic/Stearic Acid Derivatives: C6-substituted analogs exhibit 13-fold higher potency (IC50: 5.11–6.85 µM) than this compound (IC50: ~70 µM) in glioblastoma cells, likely due to enhanced lipophilicity and cellular uptake .
Binding Affinity and Docking Studies
- This compound: ΔGbind = -6.67 kcal/mol; RMSD = 0.14 Å in docking studies, forming hydrogen bonds with Glu118 and hydrophobic interactions with Trp127, Leu214, and Arg119 .
- Derivatives M4 and M11: ΔGbind = -8.63 and -8.01 kcal/mol, respectively. M11 introduces a π-cationic interaction with Arg119, improving binding .
Mechanistic Comparisons
- This compound vs. S-Trityl-Cysteine: Both inhibit Eg5, but S-trityl-cysteine lacks this compound’s off-target calcium channel modulation, which causes transient cytoskeletal changes in A549 cells .
- Fluorastrol (Class II Inhibitor): Binds Eg5 with a distinct pose but shares dependence on the 3-hydroxyl group for activity .
Key Data Tables
Table 1: Eg5 Inhibitory Activity of this compound and Derivatives
Compound | Eg5 Inhibition (%) at 10 µM | IC50 (µM) | ΔGbind (kcal/mol) | Key Structural Feature |
---|---|---|---|---|
This compound | 60% | 5.2 | -6.67 | 3-Hydroxyphenyl, thioxo |
Compound 5 | 50% | 2.37 | N/A | Modified scaffolding |
LaSOM 65 | N/A | N/A | N/A | 3-Nitrophenyl substituent |
Palmitic Deriv. | N/A | 5.11 | N/A | C6 fatty acid chain |
M11 | N/A | N/A | -8.01 | Enhanced π-cationic bonding |
Table 2: Cytotoxicity in Cancer Cell Lines
Compound | HeLa (IC50, µM) | MCF-7 (IC50, µM) | Selectivity Notes |
---|---|---|---|
This compound | 409.07 | >100 | Low neuronal toxicity |
Oxo-Monastrol | >25 | >25 | Cytostatic, not cytotoxic |
M8 | ~40 | N/A | HeLa-specific activity |
Fluorescent 8f | N/A | 8.5 | Comparable to cisplatin |
Research Findings and Implications
- Structural Requirements: The 3-hydroxyl group and thioxo moiety are indispensable for Eg5 inhibition. Modifications at R4 (e.g., fatty acid chains) enhance potency but may alter pharmacokinetics .
- Off-Target Effects: this compound’s calcium channel activity complicates its use, whereas S-trityl-cysteine and fluorastrol are more specific .
- Resistance Mechanisms: Cellular resistance emerges in compounds with superior IC50s (e.g., some derivatives in ), highlighting the need for balanced potency and stability .
Biological Activity
Monastrol is a small, cell-permeable compound recognized for its role as an allosteric inhibitor of the kinesin Eg5, which is critical for mitotic spindle formation during cell division. This article delves into the biological activity of this compound, highlighting its mechanisms of action, potential therapeutic applications, and relevant research findings.
This compound specifically targets Eg5, a member of the kinesin-5 family, which is essential for the proper functioning of the mitotic spindle. The compound inhibits both basal and microtubule-stimulated ATPase activity of Eg5 without competing with ATP binding. Instead, it appears to stabilize a conformation that alters ATP hydrolysis dynamics, leading to reduced force generation necessary for spindle formation .
Key Mechanisms:
- Allosteric Inhibition : this compound binds to an allosteric site on the Eg5 motor domain, preventing effective interaction with microtubules .
- Cell Cycle Arrest : By inhibiting Eg5, this compound causes cells to arrest in the mitotic phase, leading to a characteristic "mono-astral" spindle phenotype .
Research Findings
Numerous studies have investigated the biological activity and therapeutic potential of this compound. Below are some significant findings:
Case Studies
- Axonal Regeneration : A study by Xu et al. highlighted that this compound not only inhibits Eg5 but also enhances axonal regeneration in models of spinal cord injury. This suggests potential applications beyond oncology, possibly in neuroregenerative therapies .
- Antitumor Activity : Research has shown that this compound effectively induces cell cycle arrest and apoptosis in various cancer types. It has been noted for its selective toxicity towards proliferating cells without causing neurotoxicity associated with traditional chemotherapeutics .
Q & A
Basic Research Questions
Q. How can researchers experimentally assess Monastrol’s antiproliferative effects in vitro?
- Methodology : Use cell viability assays (e.g., MTT or Trypan Blue exclusion) on tumor and non-tumor cell lines. For example, treat MCF-7 (adenocarcinoma) and HB4a (non-tumor mammary epithelial cells) with varying this compound concentrations (e.g., 10–100 μM) and measure viability over 24–72 hours. Monitor mitotic index via immunofluorescence staining for spindle defects (e.g., monopolar spindles) .
- Key Considerations : Include controls for solvent effects (e.g., DMSO) and validate results with flow cytometry to quantify cell cycle arrest (G2/M phase) .
Q. What experimental models are suitable for studying this compound’s mechanism of action on Eg5?
- Methodology : Utilize in vitro kinesin motility assays (e.g., ATPase activity assays) and cellular models (HeLa, MCF-7) to observe spindle dynamics. Combine with siRNA knockdown of Eg5 to confirm specificity. Structural studies (e.g., X-ray crystallography) can map this compound’s allosteric binding to Eg5 .
- Data Interpretation : Compare IC50 values across cell lines to assess potency variations. Note that this compound’s efficacy is concentration-dependent and varies by cell type .
Q. How do researchers address contradictory findings on this compound’s selectivity for tumor vs. non-tumor cells?
- Methodology : Replicate studies using identical cell lines and treatment conditions. For example, HB4a cells show reduced sensitivity to this compound at lower concentrations (≤50 μM), but higher doses (100 μM) induce cytotoxicity. Cross-validate with gene expression profiling (e.g., qPCR for CDKN1A/CDKN1C) to identify differential responses .
- Analysis : Use dose-response curves and statistical tests (e.g., ANOVA) to quantify selectivity thresholds.
Advanced Research Questions
Q. What ethical guidelines apply to in vivo studies of this compound derivatives?
- Compliance : Follow ARRIVE 2.0 guidelines for animal studies, including randomization, blinding, and power analysis for sample size. Obtain approval from institutional animal care committees (IACUC) .
Properties
IUPAC Name |
ethyl 4-(3-hydroxyphenyl)-6-methyl-2-sulfanylidene-3,4-dihydro-1H-pyrimidine-5-carboxylate | |
---|---|---|
Source | PubChem | |
URL | https://pubchem.ncbi.nlm.nih.gov | |
Description | Data deposited in or computed by PubChem | |
InChI |
InChI=1S/C14H16N2O3S/c1-3-19-13(18)11-8(2)15-14(20)16-12(11)9-5-4-6-10(17)7-9/h4-7,12,17H,3H2,1-2H3,(H2,15,16,20) | |
Source | PubChem | |
URL | https://pubchem.ncbi.nlm.nih.gov | |
Description | Data deposited in or computed by PubChem | |
InChI Key |
LOBCDGHHHHGHFA-UHFFFAOYSA-N | |
Source | PubChem | |
URL | https://pubchem.ncbi.nlm.nih.gov | |
Description | Data deposited in or computed by PubChem | |
Canonical SMILES |
CCOC(=O)C1=C(NC(=S)NC1C2=CC(=CC=C2)O)C | |
Source | PubChem | |
URL | https://pubchem.ncbi.nlm.nih.gov | |
Description | Data deposited in or computed by PubChem | |
Molecular Formula |
C14H16N2O3S | |
Source | PubChem | |
URL | https://pubchem.ncbi.nlm.nih.gov | |
Description | Data deposited in or computed by PubChem | |
DSSTOX Substance ID |
DTXSID10388124, DTXSID501317756 | |
Record name | Monastrol | |
Source | EPA DSSTox | |
URL | https://comptox.epa.gov/dashboard/DTXSID10388124 | |
Description | DSSTox provides a high quality public chemistry resource for supporting improved predictive toxicology. | |
Record name | (±)-Monastrol | |
Source | EPA DSSTox | |
URL | https://comptox.epa.gov/dashboard/DTXSID501317756 | |
Description | DSSTox provides a high quality public chemistry resource for supporting improved predictive toxicology. | |
Molecular Weight |
292.36 g/mol | |
Source | PubChem | |
URL | https://pubchem.ncbi.nlm.nih.gov | |
Description | Data deposited in or computed by PubChem | |
CAS No. |
329689-23-8, 254753-54-3 | |
Record name | (±)-Monastrol | |
Source | CAS Common Chemistry | |
URL | https://commonchemistry.cas.org/detail?cas_rn=329689-23-8 | |
Description | CAS Common Chemistry is an open community resource for accessing chemical information. Nearly 500,000 chemical substances from CAS REGISTRY cover areas of community interest, including common and frequently regulated chemicals, and those relevant to high school and undergraduate chemistry classes. This chemical information, curated by our expert scientists, is provided in alignment with our mission as a division of the American Chemical Society. | |
Explanation | The data from CAS Common Chemistry is provided under a CC-BY-NC 4.0 license, unless otherwise stated. | |
Record name | Monastrol | |
Source | EPA DSSTox | |
URL | https://comptox.epa.gov/dashboard/DTXSID10388124 | |
Description | DSSTox provides a high quality public chemistry resource for supporting improved predictive toxicology. | |
Record name | (±)-Monastrol | |
Source | EPA DSSTox | |
URL | https://comptox.epa.gov/dashboard/DTXSID501317756 | |
Description | DSSTox provides a high quality public chemistry resource for supporting improved predictive toxicology. | |
Retrosynthesis Analysis
AI-Powered Synthesis Planning: Our tool employs the Template_relevance Pistachio, Template_relevance Bkms_metabolic, Template_relevance Pistachio_ringbreaker, Template_relevance Reaxys, Template_relevance Reaxys_biocatalysis model, leveraging a vast database of chemical reactions to predict feasible synthetic routes.
One-Step Synthesis Focus: Specifically designed for one-step synthesis, it provides concise and direct routes for your target compounds, streamlining the synthesis process.
Accurate Predictions: Utilizing the extensive PISTACHIO, BKMS_METABOLIC, PISTACHIO_RINGBREAKER, REAXYS, REAXYS_BIOCATALYSIS database, our tool offers high-accuracy predictions, reflecting the latest in chemical research and data.
Strategy Settings
Precursor scoring | Relevance Heuristic |
---|---|
Min. plausibility | 0.01 |
Model | Template_relevance |
Template Set | Pistachio/Bkms_metabolic/Pistachio_ringbreaker/Reaxys/Reaxys_biocatalysis |
Top-N result to add to graph | 6 |
Feasible Synthetic Routes
Disclaimer and Information on In-Vitro Research Products
Please be aware that all articles and product information presented on BenchChem are intended solely for informational purposes. The products available for purchase on BenchChem are specifically designed for in-vitro studies, which are conducted outside of living organisms. In-vitro studies, derived from the Latin term "in glass," involve experiments performed in controlled laboratory settings using cells or tissues. It is important to note that these products are not categorized as medicines or drugs, and they have not received approval from the FDA for the prevention, treatment, or cure of any medical condition, ailment, or disease. We must emphasize that any form of bodily introduction of these products into humans or animals is strictly prohibited by law. It is essential to adhere to these guidelines to ensure compliance with legal and ethical standards in research and experimentation.