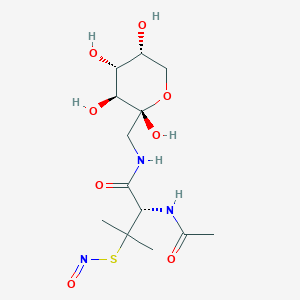
Fructose-1-SNAP
Overview
Description
Fructose-1-SNAP is a novel fructose-conjugated S-nitroso nitric oxide donor. . The synthesis of this compound represents an advancement in the development of nitric oxide donors, which are crucial for various biological processes.
Mechanism of Action
Target of Action
Fructose-1-SNAP primarily targets the enzyme Fructose-1,6-bisphosphatase (FBPase) . FBPase is a rate-controlling enzyme of gluconeogenesis and has emerged as an important target for the treatment of type 2 diabetes due to the well-recognized role of excessive endogenous glucose production (EGP) in the hyperglycemia characteristic of the disease .
Mode of Action
This compound interacts with its target, FBPase, by inhibiting its activity . This inhibition is achieved by targeting the allosteric site of the enzyme . The interaction results in a reduced rate of gluconeogenesis and EGP .
Biochemical Pathways
This compound affects the gluconeogenesis pathway . By inhibiting FBPase, it reduces the conversion of fructose-1,6-bisphosphate to fructose-6-phosphate, a key step in gluconeogenesis . This results in a decrease in endogenous glucose production, thereby reducing hyperglycemia .
Pharmacokinetics
Studies on similar compounds suggest that a high dose may be required to achieve an effective plasma level . Furthermore, the compound may be partly depleted during absorption, possibly consumed by the intestinal epithelium cells .
Result of Action
The inhibition of FBPase by this compound leads to the amelioration of both fasting and postprandial hyperglycemia without weight gain, incidence of hypoglycemia, or major perturbation of lactate or lipid homeostasis . This suggests that this compound could be a potential therapeutic agent for managing type 2 diabetes .
Action Environment
The action of this compound can be influenced by environmental factors such as temperature . For instance, the enzyme FBPase plays a crucial role in thermal homeostasis, especially under cold stress . Therefore, the environmental temperature could potentially influence the efficacy and stability of this compound.
Preparation Methods
Synthetic Routes and Reaction Conditions: The synthesis of Fructose-1-SNAP involves the conjugation of fructose with S-nitroso-N-acetylpenicillamine (SNAP). The reaction typically requires mild acidic conditions to facilitate the formation of the S-nitroso group. The process involves the following steps:
- Dissolution of fructose in an aqueous solution.
- Addition of S-nitroso-N-acetylpenicillamine to the solution.
- Stirring the mixture under controlled temperature and pH conditions to ensure complete reaction.
- Purification of the product using chromatographic techniques to obtain pure this compound.
Industrial Production Methods: Industrial production of this compound follows similar synthetic routes but on a larger scale. The process involves:
- Large-scale dissolution of fructose in water.
- Addition of S-nitroso-N-acetylpenicillamine under controlled conditions.
- Continuous monitoring of temperature and pH to optimize yield.
- Use of industrial-scale chromatography for purification and isolation of the final product.
Chemical Reactions Analysis
Types of Reactions: Fructose-1-SNAP undergoes various chemical reactions, including:
Oxidation: The S-nitroso group can be oxidized to form disulfides.
Reduction: The compound can be reduced to release nitric oxide, which is a key signaling molecule in biological systems.
Substitution: The S-nitroso group can be substituted with other nucleophiles under specific conditions.
Common Reagents and Conditions:
Oxidation: Hydrogen peroxide or other oxidizing agents.
Reduction: Reducing agents such as ascorbic acid or glutathione.
Substitution: Nucleophiles like thiols or amines under mild acidic or basic conditions.
Major Products Formed:
Oxidation: Disulfides and other oxidized derivatives.
Reduction: Nitric oxide and the corresponding reduced sugar.
Substitution: Various substituted derivatives depending on the nucleophile used.
Scientific Research Applications
Fructose-1-SNAP has a wide range of applications in scientific research:
Comparison with Similar Compounds
Fructose-1-SNAP is unique compared to other nitric oxide donors due to its fructose conjugation, which enhances its stability and bioavailability. Similar compounds include:
S-nitroso-N-acetylpenicillamine (SNAP): A widely used nitric oxide donor but lacks the stability provided by fructose conjugation.
S-nitrosoglutathione (GSNO): Another nitric oxide donor with different stability and release characteristics.
S-nitroso-N-acetyl-D,L-penicillamine (SNAP-DL): Similar to SNAP but with different stereochemistry
This compound stands out due to its enhanced stability and targeted delivery of nitric oxide, making it a valuable tool in both research and potential therapeutic applications.
Properties
IUPAC Name |
(2S)-2-acetamido-3-methyl-3-nitrososulfanyl-N-[[(2R,3S,4R,5R)-2,3,4,5-tetrahydroxyoxan-2-yl]methyl]butanamide | |
---|---|---|
Source | PubChem | |
URL | https://pubchem.ncbi.nlm.nih.gov | |
Description | Data deposited in or computed by PubChem | |
InChI |
InChI=1S/C13H23N3O8S/c1-6(17)15-9(12(2,3)25-16-23)11(21)14-5-13(22)10(20)8(19)7(18)4-24-13/h7-10,18-20,22H,4-5H2,1-3H3,(H,14,21)(H,15,17)/t7-,8-,9+,10+,13-/m1/s1 | |
Source | PubChem | |
URL | https://pubchem.ncbi.nlm.nih.gov | |
Description | Data deposited in or computed by PubChem | |
InChI Key |
PZUYSUGHXUBVOG-JJKYCLHCSA-N | |
Source | PubChem | |
URL | https://pubchem.ncbi.nlm.nih.gov | |
Description | Data deposited in or computed by PubChem | |
Canonical SMILES |
CC(=O)NC(C(=O)NCC1(C(C(C(CO1)O)O)O)O)C(C)(C)SN=O | |
Source | PubChem | |
URL | https://pubchem.ncbi.nlm.nih.gov | |
Description | Data deposited in or computed by PubChem | |
Isomeric SMILES |
CC(=O)N[C@@H](C(=O)NC[C@@]1([C@H]([C@@H]([C@@H](CO1)O)O)O)O)C(C)(C)SN=O | |
Source | PubChem | |
URL | https://pubchem.ncbi.nlm.nih.gov | |
Description | Data deposited in or computed by PubChem | |
Molecular Formula |
C13H23N3O8S | |
Source | PubChem | |
URL | https://pubchem.ncbi.nlm.nih.gov | |
Description | Data deposited in or computed by PubChem | |
DSSTOX Substance ID |
DTXSID80438746 | |
Record name | Fructose-1-SNAP | |
Source | EPA DSSTox | |
URL | https://comptox.epa.gov/dashboard/DTXSID80438746 | |
Description | DSSTox provides a high quality public chemistry resource for supporting improved predictive toxicology. | |
Molecular Weight |
381.40 g/mol | |
Source | PubChem | |
URL | https://pubchem.ncbi.nlm.nih.gov | |
Description | Data deposited in or computed by PubChem | |
CAS No. |
330688-79-4 | |
Record name | Fructose-1-SNAP | |
Source | EPA DSSTox | |
URL | https://comptox.epa.gov/dashboard/DTXSID80438746 | |
Description | DSSTox provides a high quality public chemistry resource for supporting improved predictive toxicology. | |
Retrosynthesis Analysis
AI-Powered Synthesis Planning: Our tool employs the Template_relevance Pistachio, Template_relevance Bkms_metabolic, Template_relevance Pistachio_ringbreaker, Template_relevance Reaxys, Template_relevance Reaxys_biocatalysis model, leveraging a vast database of chemical reactions to predict feasible synthetic routes.
One-Step Synthesis Focus: Specifically designed for one-step synthesis, it provides concise and direct routes for your target compounds, streamlining the synthesis process.
Accurate Predictions: Utilizing the extensive PISTACHIO, BKMS_METABOLIC, PISTACHIO_RINGBREAKER, REAXYS, REAXYS_BIOCATALYSIS database, our tool offers high-accuracy predictions, reflecting the latest in chemical research and data.
Strategy Settings
Precursor scoring | Relevance Heuristic |
---|---|
Min. plausibility | 0.01 |
Model | Template_relevance |
Template Set | Pistachio/Bkms_metabolic/Pistachio_ringbreaker/Reaxys/Reaxys_biocatalysis |
Top-N result to add to graph | 6 |
Feasible Synthetic Routes
Disclaimer and Information on In-Vitro Research Products
Please be aware that all articles and product information presented on BenchChem are intended solely for informational purposes. The products available for purchase on BenchChem are specifically designed for in-vitro studies, which are conducted outside of living organisms. In-vitro studies, derived from the Latin term "in glass," involve experiments performed in controlled laboratory settings using cells or tissues. It is important to note that these products are not categorized as medicines or drugs, and they have not received approval from the FDA for the prevention, treatment, or cure of any medical condition, ailment, or disease. We must emphasize that any form of bodily introduction of these products into humans or animals is strictly prohibited by law. It is essential to adhere to these guidelines to ensure compliance with legal and ethical standards in research and experimentation.