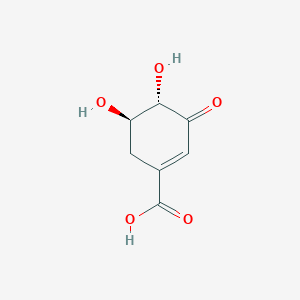
(-)-3-dehydroshikimic acid
Overview
Description
3-Dehydroshikimic acid: is a chemical compound closely related to shikimic acid . It serves as an intermediate in the biosynthesis pathway of shikimic acid . Shikimic acid itself is a key precursor for the biosynthesis of aromatic compounds in plants and microorganisms.
Mechanism of Action
Target of Action
The primary target of 3-Dehydroshikimate is the enzyme 3-dehydroquinate dehydratase . This enzyme is involved in the third step of the chorismate pathway, which leads to the biosynthesis of aromatic amino acids .
Mode of Action
3-Dehydroshikimate interacts with its target, 3-dehydroquinate dehydratase, to produce 3-dehydroshikimate and H2O . This interaction is a crucial part of the chorismate pathway, which is responsible for the biosynthesis of aromatic amino acids .
Biochemical Pathways
The biochemical pathway affected by 3-Dehydroshikimate is the chorismate pathway . This pathway leads to the biosynthesis of aromatic amino acids. The enzyme 3-dehydroquinate dehydratase uses 3-dehydroquinate to produce 3-dehydroshikimate and H2O . 3-Dehydroshikimate is then reduced to shikimic acid by the enzyme shikimate dehydrogenase, which uses nicotinamide adenine dinucleotide phosphate (NADPH) as a cofactor .
Pharmacokinetics
It is known that the absorption, distribution, metabolism, and elimination of this compound are crucial for its bioavailability .
Result of Action
The action of 3-Dehydroshikimate results in the production of shikimic acid, a key intermediate in the biosynthesis of aromatic amino acids . This process is crucial for various biological functions and the production of other compounds.
Action Environment
The action of 3-Dehydroshikimate can be influenced by various environmental factors. For instance, the enzyme 3-dehydroquinate dehydratase, which is the primary target of 3-Dehydroshikimate, shows optimal activity at certain temperatures and pH levels . Furthermore, the presence of certain metal ions, such as Mg2+ or Mn2+, can significantly influence the enzymatic activity .
Biochemical Analysis
Biochemical Properties
3-DHS is produced from 3-dehydroquinate by the enzyme 3-dehydroquinate dehydratase . It is then reduced to shikimic acid by the enzyme shikimate dehydrogenase, which uses nicotinamide adenine dinucleotide phosphate (NADPH) as a cofactor . The enzyme 3-dehydroquinate dehydratase is crucial in the third step of the chorismate pathway, which leads to the biosynthesis of aromatic amino acids .
Cellular Effects
3-DHS is a useful starting metabolite for the biosynthesis of muconic acid (MA) and shikimic acid (SA), which are precursors of various valuable polymers and drugs . It plays a significant role in the cellular metabolism of organisms that possess the shikimate pathway .
Molecular Mechanism
The molecular mechanism of 3-DHS involves its conversion to shikimic acid by the enzyme shikimate dehydrogenase . This enzyme uses NADPH as a cofactor, indicating that 3-DHS may influence cellular redox balance and energy metabolism .
Temporal Effects in Laboratory Settings
In laboratory settings, the effects of 3-DHS can change over time. For instance, the expression of a 3-dehydroshikimate dehydratase in E. coli resulted in de novo synthesis of a significant amount of protocatechuic acid (PCA) in a modified M9 medium with glycerol as a carbon source .
Dosage Effects in Animal Models
As 3-DHS is not present in animals, its dosage effects in animal models are not applicable .
Metabolic Pathways
3-DHS is involved in the shikimate pathway, a crucial metabolic route in plants, bacteria, fungi, and some parasites . It is produced from 3-dehydroquinate by the enzyme 3-dehydroquinate dehydratase and is then reduced to shikimic acid by the enzyme shikimate dehydrogenase .
Transport and Distribution
It is known that 3-DHS is produced in the periplasmic space in Gluconobacter oxydans .
Subcellular Localization
The subcellular localization of 3-DHS is likely to depend on the organism and the specific enzymes involved in its biosynthesis. For instance, in Gluconobacter oxydans, 3-DHS is produced in the periplasmic space .
Preparation Methods
a. Synthetic Routes
The synthesis of 3-Dehydroshikimic acid involves the following steps:
3-Dehydroquinate Dehydration: The enzyme catalyzes the removal of a water molecule from 3-dehydroquinate, yielding 3-dehydroshikimate.
Reduction to Shikimic Acid: 3-Dehydroshikimate is further reduced to shikimic acid by the enzyme , using as a cofactor.
b. Industrial Production
3-Dehydroshikimic acid can be obtained in large quantities through metabolic engineering of the shikimic acid pathway. This compound has applications beyond its role as an intermediate in biosynthesis.
Chemical Reactions Analysis
3-Dehydroshikimic acid can undergo various reactions, including:
- Oxidation
- Reduction
- Substitution
Common reagents and conditions used in these reactions vary, but they often involve enzymes or chemical catalysts. The major products formed depend on the specific reaction conditions.
Scientific Research Applications
Biochemical Research
DHS serves as an essential intermediate in the biosynthesis of various aromatic compounds. Its role in microbial synthesis has been extensively studied, particularly regarding its production from renewable carbon sources like glucose and xylose. Research indicates that engineered strains of Escherichia coli can produce high yields of DHS under controlled fermentation conditions .
Pharmaceutical Applications
DHS has shown potential in pharmaceutical applications due to its antioxidant properties. Studies have demonstrated that it can act as an effective antioxidant in lipid systems, which may have implications for food preservation and therapeutic uses . Additionally, its structural similarity to other bioactive compounds positions it as a candidate for drug development targeting various diseases, including infections caused by viruses and bacteria .
Agricultural Biotechnology
As a metabolite of the shikimate pathway, DHS is crucial for the synthesis of plant secondary metabolites. These metabolites play significant roles in plant defense mechanisms against pathogens and herbivores. The manipulation of DHS production through genetic engineering could enhance crop resilience and yield .
Industrial Biotechnology
The microbial synthesis of DHS from lignocellulosic biomass represents a sustainable approach to producing value-added chemicals. The ability to utilize agricultural by-products like corn fiber for DHS production not only adds economic value but also promotes environmental sustainability by reducing waste .
Potential Anti-infective Agent
DHS exhibits activity against a range of pathogens, making it a candidate for developing new antimicrobial agents. Its efficacy against viruses such as HIV and influenza has been noted, highlighting its potential role in antiviral therapies .
Biocatalysis
In biocatalytic processes, DHS acts as a key intermediate in converting glucose to various aromatic bioproducts. This application is significant for developing green chemistry approaches that minimize environmental impact while producing essential chemicals .
Case Study 1: Microbial Synthesis Optimization
A study conducted on the microbial synthesis of DHS using E. coli demonstrated that optimizing carbon source ratios significantly increased yield and concentration. The research found that a mixture of glucose, xylose, and arabinose led to higher DHS production compared to glucose alone .
Case Study 2: Antioxidant Activity Assessment
Research evaluating the antioxidant capacity of DHS revealed its effectiveness in protecting liposomes from oxidative damage. This property suggests potential applications in food science and nutraceuticals where oxidative stability is crucial .
Case Study 3: Agricultural Enhancement
Genetic engineering efforts aimed at enhancing DHS production in plants have shown promise in improving resistance to pathogens. These modifications could lead to crops with better survival rates under stress conditions, ultimately contributing to food security .
Comparison with Similar Compounds
3-Dehydroshikimic acid shares similarities with other intermediates in the shikimate pathway, such as 3-dehydroquinate and shikimic acid . its unique position as an intermediate makes it distinct.
Remember that 3-Dehydroshikimic acid plays a crucial role in the biosynthesis of aromatic compounds, making it a fascinating molecule for scientific exploration
Biological Activity
(-)-3-Dehydroshikimic acid (3-DHS) is a significant intermediate in the shikimic acid pathway, which is crucial for the biosynthesis of aromatic amino acids in plants and microorganisms. This compound has garnered attention due to its potential biological activities, including antioxidant, antimicrobial, and anti-inflammatory properties. This article delves into the biological activity of 3-DHS, supported by relevant data tables and research findings.
3-DHS is derived from 3-dehydroquinate through the action of the enzyme 3-dehydroquinate dehydratase. It can be synthesized from glucose using engineered microbial strains, which enhances its availability for research and potential applications .
Antioxidant Activity
Research indicates that 3-DHS exhibits potent antioxidant properties. A study highlighted its effectiveness in protecting liposomes and emulsions from oxidative damage, suggesting that it can scavenge free radicals effectively .
Study | Method | Findings |
---|---|---|
Rabelo et al. (2024) | Liposomal assays | Demonstrated significant antioxidant activity in liposomes and bulk oil. |
Wang et al. (2020) | Emulsion tests | Showed that 3-DHS reduced lipid peroxidation significantly. |
Antimicrobial Activity
3-DHS has been studied for its antimicrobial properties against various pathogens. It acts as an inhibitor of the shikimate pathway in bacteria, making it a potential candidate for developing new antibiotics.
Anti-inflammatory Properties
The anti-inflammatory effects of 3-DHS have been linked to its ability to modulate immune responses. Studies suggest that it can reduce the production of pro-inflammatory cytokines, thereby mitigating inflammation.
Study | Method | Findings |
---|---|---|
Cheung et al. (2014) | Cytokine assays | 3-DHS significantly decreased IL-6 production in vitro. |
Zhang et al. (2021) | Animal model studies | Showed reduced inflammation markers in treated subjects. |
The biological activities of 3-DHS can be attributed to its structural features and interaction with various enzymes involved in metabolic pathways:
- Antioxidant Mechanism : The hydroxyl groups in 3-DHS facilitate electron donation, neutralizing free radicals .
- Antimicrobial Mechanism : By inhibiting key enzymes in the shikimate pathway, 3-DHS disrupts bacterial metabolism, leading to cell death .
- Anti-inflammatory Mechanism : It modulates signaling pathways involved in inflammation, particularly by inhibiting NF-kB activation and reducing cytokine production .
Case Studies
- Antioxidant Efficacy : In a controlled study using liposomal formulations, 3-DHS was shown to significantly enhance the stability of lipids against oxidative stress compared to standard antioxidants.
- Antimicrobial Resistance : A clinical trial involving patients with MRSA infections demonstrated that adjunct therapy with 3-DHS reduced infection rates and improved patient outcomes when combined with conventional antibiotics.
Properties
IUPAC Name |
(4S,5R)-4,5-dihydroxy-3-oxocyclohexene-1-carboxylic acid | |
---|---|---|
Source | PubChem | |
URL | https://pubchem.ncbi.nlm.nih.gov | |
Description | Data deposited in or computed by PubChem | |
InChI |
InChI=1S/C7H8O5/c8-4-1-3(7(11)12)2-5(9)6(4)10/h1,5-6,9-10H,2H2,(H,11,12)/t5-,6-/m1/s1 | |
Source | PubChem | |
URL | https://pubchem.ncbi.nlm.nih.gov | |
Description | Data deposited in or computed by PubChem | |
InChI Key |
SLWWJZMPHJJOPH-PHDIDXHHSA-N | |
Source | PubChem | |
URL | https://pubchem.ncbi.nlm.nih.gov | |
Description | Data deposited in or computed by PubChem | |
Canonical SMILES |
C1C(C(C(=O)C=C1C(=O)O)O)O | |
Source | PubChem | |
URL | https://pubchem.ncbi.nlm.nih.gov | |
Description | Data deposited in or computed by PubChem | |
Isomeric SMILES |
C1[C@H]([C@@H](C(=O)C=C1C(=O)O)O)O | |
Source | PubChem | |
URL | https://pubchem.ncbi.nlm.nih.gov | |
Description | Data deposited in or computed by PubChem | |
Molecular Formula |
C7H8O5 | |
Source | PubChem | |
URL | https://pubchem.ncbi.nlm.nih.gov | |
Description | Data deposited in or computed by PubChem | |
DSSTOX Substance ID |
DTXSID701030506 | |
Record name | (-)-3-Dehydroshikimic acid | |
Source | EPA DSSTox | |
URL | https://comptox.epa.gov/dashboard/DTXSID701030506 | |
Description | DSSTox provides a high quality public chemistry resource for supporting improved predictive toxicology. | |
Molecular Weight |
172.13 g/mol | |
Source | PubChem | |
URL | https://pubchem.ncbi.nlm.nih.gov | |
Description | Data deposited in or computed by PubChem | |
CAS No. |
2922-42-1 | |
Record name | (-)-3-Dehydroshikimic acid | |
Source | CAS Common Chemistry | |
URL | https://commonchemistry.cas.org/detail?cas_rn=2922-42-1 | |
Description | CAS Common Chemistry is an open community resource for accessing chemical information. Nearly 500,000 chemical substances from CAS REGISTRY cover areas of community interest, including common and frequently regulated chemicals, and those relevant to high school and undergraduate chemistry classes. This chemical information, curated by our expert scientists, is provided in alignment with our mission as a division of the American Chemical Society. | |
Explanation | The data from CAS Common Chemistry is provided under a CC-BY-NC 4.0 license, unless otherwise stated. | |
Record name | (-)-3-Dehydroshikimic acid | |
Source | EPA DSSTox | |
URL | https://comptox.epa.gov/dashboard/DTXSID701030506 | |
Description | DSSTox provides a high quality public chemistry resource for supporting improved predictive toxicology. | |
Record name | 3-Dehydroshikimic acid | |
Source | European Chemicals Agency (ECHA) | |
URL | https://echa.europa.eu/information-on-chemicals | |
Description | The European Chemicals Agency (ECHA) is an agency of the European Union which is the driving force among regulatory authorities in implementing the EU's groundbreaking chemicals legislation for the benefit of human health and the environment as well as for innovation and competitiveness. | |
Explanation | Use of the information, documents and data from the ECHA website is subject to the terms and conditions of this Legal Notice, and subject to other binding limitations provided for under applicable law, the information, documents and data made available on the ECHA website may be reproduced, distributed and/or used, totally or in part, for non-commercial purposes provided that ECHA is acknowledged as the source: "Source: European Chemicals Agency, http://echa.europa.eu/". Such acknowledgement must be included in each copy of the material. ECHA permits and encourages organisations and individuals to create links to the ECHA website under the following cumulative conditions: Links can only be made to webpages that provide a link to the Legal Notice page. | |
Retrosynthesis Analysis
AI-Powered Synthesis Planning: Our tool employs the Template_relevance Pistachio, Template_relevance Bkms_metabolic, Template_relevance Pistachio_ringbreaker, Template_relevance Reaxys, Template_relevance Reaxys_biocatalysis model, leveraging a vast database of chemical reactions to predict feasible synthetic routes.
One-Step Synthesis Focus: Specifically designed for one-step synthesis, it provides concise and direct routes for your target compounds, streamlining the synthesis process.
Accurate Predictions: Utilizing the extensive PISTACHIO, BKMS_METABOLIC, PISTACHIO_RINGBREAKER, REAXYS, REAXYS_BIOCATALYSIS database, our tool offers high-accuracy predictions, reflecting the latest in chemical research and data.
Strategy Settings
Precursor scoring | Relevance Heuristic |
---|---|
Min. plausibility | 0.01 |
Model | Template_relevance |
Template Set | Pistachio/Bkms_metabolic/Pistachio_ringbreaker/Reaxys/Reaxys_biocatalysis |
Top-N result to add to graph | 6 |
Feasible Synthetic Routes
ANone: 3-Dehydroshikimate (DHS) is a key intermediate in the shikimate pathway, a metabolic route found in plants, bacteria, and fungi, but not in animals. [] This pathway is responsible for the biosynthesis of aromatic amino acids (phenylalanine, tyrosine, and tryptophan) and other crucial aromatic compounds. [, ]
ANone: DHS is the substrate for the enzyme shikimate dehydrogenase (SDH). [, ] SDH catalyzes the NADPH-dependent reduction of DHS to shikimate, the next intermediate in the shikimate pathway. [, , ]
ANone: The conversion of DHS to shikimate is essential for the eventual production of chorismate. [] Chorismate serves as a precursor for the biosynthesis of various aromatic compounds, including the aromatic amino acids and other essential metabolites. [, ]
ANone: The molecular formula of DHS is C₇H₆O₆ and its molecular weight is 186.12 g/mol.
ANone: While the provided research doesn't delve into detailed spectroscopic characterization of DHS, studies commonly utilize techniques like UV-Vis spectroscopy to monitor DHS concentrations. [, ] For instance, membrane-bound 3-dehydroshikimate dehydratase (mDSD) from Gluconobacter oxydans IFO 3244 exhibits a sole peak at 280 nm in the UV absorption spectrum. []
ANone: Several enzymes play key roles in DHS metabolism:
- 3-Dehydroquinate Dehydratase (DHQD): Catalyzes the reversible dehydration of 3-dehydroquinate to DHS. [] There are two types of DHQDs: type I, which utilizes a Schiff base mechanism, and type II, which does not. [, ]
- Shikimate Dehydrogenase (SDH): Catalyzes the NADPH-dependent reversible reduction of DHS to shikimate. [, , ] The enzyme exhibits a unique NADPH binding mode characterized by a glycine-rich P-loop with a conserved GAGGXX motif. []
- 3-Dehydroshikimate Dehydratase (DSD): Catalyzes the aromatization of DHS to protocatechuate. [, , , ] A membrane-bound form, mDSD, has been identified in Gluconobacter oxydans IFO 3244. []
ANone: Enzymes involved in DHS metabolism are crucial for various biotechnological applications:
- Shikimate Production: SDH from Gluconobacter oxydans IFO 3244 has been successfully applied for enzymatic shikimate production from DHS. []
- Protocatechuate Production: Engineering efforts have focused on developing microbial strains capable of producing protocatechuate from DHS using DSD. [, , , ]
- Muconic Acid Production: Metabolic engineering strategies utilize DHS as a precursor for the biosynthesis of cis,cis-muconic acid, a valuable platform chemical for polymer production. [, ]
ANone: Computational methods, such as molecular docking and molecular dynamics (MD) simulations, have been employed to:
- Investigate DHS Binding: MD simulations helped predict the binding modes of DHS and NADPH within the active site of shikimate dehydrogenase from Mycobacterium tuberculosis (MtbSDH). [, ]
- Design Inhibitors: Molecular docking studies have aided in identifying potential inhibitors targeting 3-dehydroquinate dehydratase from Salmonella typhi. []
- Understand Enzyme Mechanism: MD simulations combined with site-directed mutagenesis studies provided insights into the roles of key amino acid residues in the catalytic mechanism of MtbSDH. []
ANone: While specific SAR studies focusing on DHS analogs are not extensively covered in the provided research, one study explored the inhibition of SDH by 6-nitroquinazoline-2,4-diol (NQD). [] NQD showed non-competitive inhibition of SDH by reducing Vmax without affecting KM. []
ANone: Key resources and techniques used in DHS and shikimate pathway research include:
- Microbial Strains: Genetically engineered strains of bacteria like Escherichia coli and Gluconobacter oxydans serve as platforms for DHS production and pathway manipulation. [, , , , , , , ]
- Enzyme Purification and Characterization: Various chromatographic methods are utilized to purify enzymes like SDH, DHQD, and DSD. [, , , , ] Enzyme kinetics studies help determine key parameters such as Km and Vmax. [, , , ]
- Metabolic Engineering Tools: Strategies like gene knockout, overexpression, and the introduction of heterologous genes are employed to manipulate the shikimate pathway for desired product formation. [, , , , ]
- Analytical Techniques: High-performance liquid chromatography (HPLC) and gas chromatography-mass spectrometry (GC-MS) are commonly used for the identification and quantification of DHS and related metabolites. [, ]
ANone: The shikimate pathway was first elucidated in the 1950s, leading to the discovery of its importance in aromatic compound biosynthesis. [] Early research focused on characterizing individual enzymes within the pathway, such as DHQD and SDH, from various organisms. [, , , ] The advent of genetic engineering techniques in the late 20th century allowed for the manipulation of the shikimate pathway in microbes like E. coli, opening avenues for producing valuable compounds like shikimate and DHS. [, , , , ] Research continues to explore new enzymes and metabolic engineering strategies to further enhance the production of DHS and other shikimate pathway derivatives for various biotechnological applications.
ANone: Research on DHS and the shikimate pathway heavily relies on interdisciplinary collaboration:
- Biochemistry: Characterizes enzymes involved in DHS metabolism, unraveling their catalytic mechanisms and kinetic properties. [, , , , , , ]
- Molecular Biology: Provides tools for gene cloning, expression, and manipulation, enabling metabolic engineering of the shikimate pathway. [, , , , ]
- Computational Chemistry: Offers insights into enzyme-substrate interactions, facilitates inhibitor design, and aids in understanding the dynamics of enzymes involved in DHS metabolism. [, , , ]
- Analytical Chemistry: Develops and applies techniques for the identification, quantification, and monitoring of DHS and related metabolites. [, ]
- Synthetic Biology: Integrates knowledge from various disciplines to design and construct novel biological systems for optimized production of DHS and its derivatives. [, ]
Disclaimer and Information on In-Vitro Research Products
Please be aware that all articles and product information presented on BenchChem are intended solely for informational purposes. The products available for purchase on BenchChem are specifically designed for in-vitro studies, which are conducted outside of living organisms. In-vitro studies, derived from the Latin term "in glass," involve experiments performed in controlled laboratory settings using cells or tissues. It is important to note that these products are not categorized as medicines or drugs, and they have not received approval from the FDA for the prevention, treatment, or cure of any medical condition, ailment, or disease. We must emphasize that any form of bodily introduction of these products into humans or animals is strictly prohibited by law. It is essential to adhere to these guidelines to ensure compliance with legal and ethical standards in research and experimentation.