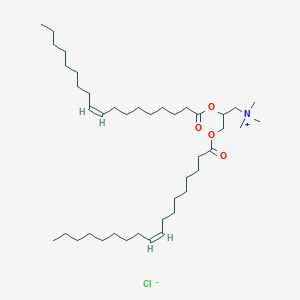
Dotap chloride
Overview
Description
1,2-Dioleoyl-3-trimethylammonium-propane chloride, commonly known as DOTAP chloride, is a cationic lipid widely used in the field of gene therapy and molecular biology. It is particularly effective for the liposomal transfection of oligonucleotides, such as DNA and RNA, into cells. The key structural element of this compound is its quaternary ammonium headgroup, which facilitates interactions with nucleic acids and cell membranes .
Preparation Methods
Synthetic Routes and Reaction Conditions
The synthesis of DOTAP chloride typically involves the esterification of 1,2-dioleoyl-sn-glycerol with 3-chloropropyltrimethylammonium chloride. The reaction is carried out under anhydrous conditions using a suitable solvent such as dichloromethane or chloroform. The reaction mixture is then purified through column chromatography to obtain the pure product .
Industrial Production Methods
In industrial settings, the production of this compound follows similar synthetic routes but on a larger scale. The process involves the use of high-purity reagents and solvents to ensure the quality of the final product. The reaction conditions are optimized to maximize yield and minimize impurities. The product is then subjected to rigorous quality control measures to ensure its suitability for use in biomedical applications .
Chemical Reactions Analysis
Chemical Interactions and Behavior
- Solubility and Hydration: DOTAP chloride readily forms stable liposomes in water, unlike DOTAP iodide, which exhibits poor solubility due to tight binding of the iodide counterion and subsequent expulsion of water [1, 4].
- Interaction with DNA: this compound is employed to transfect plasmid DNA into eukaryotic cells . Mixing DOTAP with DNA results in the formation of stable complexes that can be directly added to a cell culture .
- Influence of Counterions: The type of counterion (chloride vs. iodide) significantly affects the hydration behavior of the DOTAP headgroup [1, 4].
- Interaction with other lipids: this compound can be mixed with helper lipids, such as DOPC, to form multilayered lamellar phases .
Factors Affecting this compound Behavior
- Buffer Conditions: The zeta potential of DOTAP nanoparticles varies depending on the buffer used. For example, a higher zeta potential was observed in 5% glucose solution compared to MES-buffered saline .
- Salts: The presence of salts like sodium chloride impacts the preparation of lipid dispersions containing cationic lipid analogues .
Potential Reactions and Degradation
Scientific Research Applications
Gene Delivery Systems
Transfection Efficiency
DOTAP chloride is widely recognized for its role in facilitating the transfection of nucleic acids (DNA and RNA) into cells. It forms stable complexes with negatively charged nucleic acids through electrostatic interactions, enhancing cellular uptake via endocytosis and promoting endosomal escape.
- In Vitro Applications : Studies have shown high transfection efficiency in various cell lines when using DOTAP-based liposomes. For example, a study demonstrated that DOTAP effectively delivered siRNA for gene silencing in cancer cells, leading to significant reductions in target gene expression .
- In Vivo Applications : DOTAP has been utilized in preclinical animal models for delivering therapeutic genes. Its ability to encapsulate nucleic acids into lipid nanoparticles (LNPs) has been pivotal in developing mRNA vaccines, notably during the COVID-19 pandemic .
Vaccine Development
Lipid Nanoparticles for mRNA Vaccines
The emergence of mRNA vaccines has highlighted the importance of this compound as a key component in lipid nanoparticle formulations. Its role in stabilizing mRNA and enhancing immune responses has been crucial.
- COVID-19 Vaccines : DOTAP was integral to the formulation of mRNA vaccines, where it facilitated the delivery of mRNA encoding viral proteins, thus eliciting an immune response . The use of DOTAP in these formulations has led to improved vaccine efficacy and stability.
Pharmaceutical Formulations
Stability and Shelf Life
Recent advancements in the crystallization of this compound have resulted in stable forms suitable for pharmaceutical applications. The crystalline variants exhibit enhanced stability and longer shelf life, making them ideal for drug formulation processes.
- Pharmaceutical Compositions : this compound can be incorporated into various pharmaceutical formulations such as liposomes, microemulsions, and nanoparticles. These formulations can encapsulate active compounds including peptides, nucleotides, and cytostatic agents .
Research Applications
Modeling Biological Processes
DOTAP's unique chemical properties make it a valuable tool in research settings. It is used to study membrane interactions and cellular processes due to its ability to form stable vesicles.
- Voltage-Gated Ion Channels : Research utilizing DOTAP has provided insights into the behavior of ion channels within cellular membranes, aiding in understanding their physiological roles .
Data Tables
Application Area | Specific Use Case |
---|---|
Gene Therapy | Transfection of siRNA for cancer treatment |
Vaccine Development | Component of mRNA vaccines (e.g., COVID-19) |
Drug Delivery | Encapsulation of peptides and nucleotides |
Research | Study of membrane dynamics and ion channel behavior |
Case Studies
-
mRNA Vaccine Development
A pivotal study highlighted the use of DOTAP in formulating LNPs for mRNA vaccines against COVID-19. The incorporation of DOTAP enhanced the stability and delivery efficiency of the mRNA payload, leading to robust immune responses in clinical trials . -
Gene Silencing in Cancer Models
In a research project aimed at silencing specific oncogenes using siRNA delivered via DOTAP-liposomes, significant reductions in tumor growth were observed in mouse models, showcasing its potential as a therapeutic agent .
Mechanism of Action
The mechanism of action of DOTAP chloride involves the formation of lipoplexes with nucleic acids. The quaternary ammonium headgroup of this compound interacts with the negatively charged phosphate groups of nucleic acids, forming stable complexes. These lipoplexes are then taken up by cells through endocytosis, allowing the nucleic acids to be released into the cytoplasm and exert their effects . The molecular targets of this compound include cell membranes and nucleic acids, and the pathways involved include endocytosis and intracellular trafficking .
Comparison with Similar Compounds
Similar Compounds
1,2-Dioleoyl-3-trimethylammonium-propane (DOTAP): An ester analog of DOTAP chloride, used for similar applications in gene therapy and molecular biology.
N-[1-(2,3-Dioleoyloxy)propyl]-N,N,N-trimethylammonium chloride (DOTMA): Another cationic lipid used for the transfection of nucleic acids.
Uniqueness
This compound is unique due to its high transfection efficiency and ability to form stable lipoplexes with nucleic acids. Its quaternary ammonium headgroup provides strong interactions with nucleic acids, making it a preferred choice for gene therapy applications .
Biological Activity
Introduction
Dotap chloride, or 1,2-dioleoyl-3-trimethylammonium propane (DOTAP), is a synthetic cationic lipid widely utilized in gene delivery systems due to its ability to form lipoplexes with nucleic acids. This article explores the biological activity of this compound, focusing on its mechanisms of action, effectiveness in gene delivery, and potential therapeutic applications.
Structural Properties
DOTAP is characterized by its quaternary ammonium headgroup, which is crucial for its interaction with negatively charged nucleic acids. The lipid's structure allows it to form stable complexes with DNA or RNA, facilitating cellular uptake through endocytosis. The hydrophobic tails of DOTAP enhance membrane fusion, promoting the release of genetic material into the cytoplasm.
The transfection efficiency of DOTAP is influenced by several factors:
- Lipid Composition : The presence of cholesterol in DOTAP formulations enhances stability and transfection efficiency.
- Charge Density : Higher charge densities improve binding to nucleic acids but can also increase cytotoxicity.
- Counterions : The choice of counterion (e.g., chloride vs. iodide) affects the hydration properties and solubility of DOTAP, impacting its biological activity .
Gene Delivery
DOTAP has been extensively studied for its role in delivering plasmid DNA and mRNA. Research indicates that DOTAP-based lipoplexes can effectively transfect various cell types, including lung cancer cells. For instance, DOTAP/cholesterol lipoplexes have shown promising results in delivering the tumor suppressor gene FUS1 in non-small cell lung carcinoma models, leading to positive clinical responses .
Cytotoxicity Studies
While DOTAP is effective for gene delivery, it exhibits dose-dependent cytotoxicity. Studies have demonstrated that higher concentrations of DOTAP lead to increased cell death, particularly in non-PEGylated formulations. Optimizing the lipid-to-nucleic acid ratio and incorporating PEGylation can mitigate toxicity while maintaining transfection efficiency .
Case Studies
- Lung Cancer Treatment : In a clinical setting, DOTAP/cholesterol lipoplexes were used to deliver therapeutic genes to patients with end-stage non-small cell lung carcinoma. Positive responses were reported, highlighting the potential of DOTAP in cancer gene therapy .
- mRNA Vaccines : Recent studies have explored the use of DOTAP in mRNA vaccine formulations. The optimization of lipid ratios demonstrated enhanced transfection efficiency and reduced cytotoxicity, making DOTAP a candidate for future vaccine development .
Comparative Analysis of Formulations
The following table summarizes various formulations involving DOTAP and their respective biological activities:
Formulation | Components | Transfection Efficiency | Cytotoxicity | Application |
---|---|---|---|---|
DOTAP/Cholesterol | 50% DOTAP, 50% Cholesterol | High | Moderate | Gene therapy for lung cancer |
PEGylated DOTAP | 50% DOTAP, 50% Cholesterol + PEG | High | Low | mRNA vaccine development |
Non-PEGylated DOTAP | 100% DOTAP | Moderate | High | General gene delivery |
Q & A
Basic Research Questions
Q. What structural properties of DOTAP chloride make it effective for nucleic acid delivery?
this compound’s efficacy arises from its cationic quaternary ammonium group, which facilitates electrostatic binding to negatively charged nucleic acids. The unsaturated oleoyl chains enhance membrane fluidity, promoting cellular uptake and endosomal escape. These features are critical for forming stable liposome-nucleic acid complexes .
Q. What is the standard protocol for preparing this compound-based liposomes?
A widely used method involves dissolving this compound with a helper lipid (e.g., cholesterol or DOPE) in chloroform, evaporating the solvent to form a lipid film, and hydrating the film with an aqueous buffer. The suspension is then sonicated or extruded through polycarbonate membranes (100–200 nm pore size) to achieve uniform vesicle size. Dynamic light scattering (DLS) is recommended for validating particle size and polydispersity .
Q. How do researchers quantify the binding efficiency between this compound and nucleic acids?
Gel retardation assays are commonly employed: increasing DOTAP/nucleic acid ratios are tested, and electrophoretic mobility shifts indicate complete complex formation. Fluorescence-based methods (e.g., ethidium bromide exclusion) or zeta potential measurements (transition from negative to positive charge) provide additional validation .
Advanced Research Questions
Q. How can this compound formulations be optimized to balance transfection efficiency and cytotoxicity?
Cytotoxicity often correlates with excessive positive charge density. Researchers adjust the molar ratio of DOTAP to neutral lipids (e.g., DOPE at 1:1) to reduce toxicity while maintaining transfection efficiency. Incorporating PEGylated lipids or optimizing lipid nanoparticle (LNP) size (<150 nm) further improves biocompatibility and targeting .
Q. What experimental strategies address batch-to-batch variability in this compound-based liposome synthesis?
Variability can arise from differences in lipid oxidation or hydration conditions. Implementing strict quality control measures—such as NMR or mass spectrometry to verify lipid purity, and standardized hydration buffers (e.g., pH 7.4, 10 mM HEPES)—ensures reproducibility. Lyophilization with cryoprotectants (e.g., sucrose) also stabilizes liposomes for long-term storage .
Q. How do polymorphic crystal forms of this compound impact formulation stability?
Polymorphs (e.g., α- vs. β-crystalline phases) influence hydration kinetics and liposome uniformity. X-ray powder diffraction (XRPD) and differential scanning calorimetry (DSC) identify polymorphic transitions. Pre-formulation studies under varied temperature/humidity conditions help select stable crystal forms for scalable production .
Q. Data Analysis and Contradiction Resolution
Q. How should researchers interpret conflicting reports on this compound’s transfection efficiency across cell lines?
Discrepancies often stem from cell-specific factors (e.g., membrane composition, endocytic activity). Systematic controls, including parallel experiments with positive controls (e.g., Lipofectamine) and serum-free vs. serum-containing media, clarify context-dependent performance. Meta-analyses comparing lipid/DNA ratios and cell viability metrics across studies are recommended .
Q. What statistical approaches are appropriate for analyzing dose-response data in this compound-mediated gene delivery?
Non-linear regression models (e.g., sigmoidal dose-response curves) quantify transfection efficiency versus lipid concentration. ANOVA with post-hoc tests (e.g., Tukey’s HSD) identifies significant differences between formulations. Researchers should report effect sizes and confidence intervals to enhance reproducibility .
Q. Methodological Best Practices
Q. What characterization techniques are essential for this compound formulations?
Parameter | Technique | Key Metrics |
---|---|---|
Particle Size | Dynamic Light Scattering (DLS) | Mean diameter, PDI (<0.3 ideal) |
Surface Charge | Zeta Potential | +20 to +40 mV for stable complexes |
Structural Integrity | Transmission Electron Microscopy (TEM) | Spherical morphology, absence of aggregates |
Nucleic Acid Binding | Gel Electrophoresis | Complete complexation at optimal ratios |
These metrics ensure compliance with guidelines for reproducible nanomedicine research .
Q. How can researchers validate the biological activity of this compound-delivered nucleic acids?
Luciferase or GFP reporter assays quantify expression levels post-transfection. qRT-PCR or Western blotting confirms target gene/protein expression. For siRNA delivery, knockdown efficiency (≥70%) should be validated using RT-qPCR and functional assays (e.g., apoptosis or proliferation tests) .
Properties
IUPAC Name |
2,3-bis[[(Z)-octadec-9-enoyl]oxy]propyl-trimethylazanium;chloride | |
---|---|---|
Source | PubChem | |
URL | https://pubchem.ncbi.nlm.nih.gov | |
Description | Data deposited in or computed by PubChem | |
InChI |
InChI=1S/C42H80NO4.ClH/c1-6-8-10-12-14-16-18-20-22-24-26-28-30-32-34-36-41(44)46-39-40(38-43(3,4)5)47-42(45)37-35-33-31-29-27-25-23-21-19-17-15-13-11-9-7-2;/h20-23,40H,6-19,24-39H2,1-5H3;1H/q+1;/p-1/b22-20-,23-21-; | |
Source | PubChem | |
URL | https://pubchem.ncbi.nlm.nih.gov | |
Description | Data deposited in or computed by PubChem | |
InChI Key |
KSXTUUUQYQYKCR-LQDDAWAPSA-M | |
Source | PubChem | |
URL | https://pubchem.ncbi.nlm.nih.gov | |
Description | Data deposited in or computed by PubChem | |
Canonical SMILES |
CCCCCCCCC=CCCCCCCCC(=O)OCC(C[N+](C)(C)C)OC(=O)CCCCCCCC=CCCCCCCCC.[Cl-] | |
Source | PubChem | |
URL | https://pubchem.ncbi.nlm.nih.gov | |
Description | Data deposited in or computed by PubChem | |
Isomeric SMILES |
CCCCCCCC/C=C\CCCCCCCC(=O)OCC(C[N+](C)(C)C)OC(=O)CCCCCCC/C=C\CCCCCCCC.[Cl-] | |
Source | PubChem | |
URL | https://pubchem.ncbi.nlm.nih.gov | |
Description | Data deposited in or computed by PubChem | |
Molecular Formula |
C42H80ClNO4 | |
Source | PubChem | |
URL | https://pubchem.ncbi.nlm.nih.gov | |
Description | Data deposited in or computed by PubChem | |
Molecular Weight |
698.5 g/mol | |
Source | PubChem | |
URL | https://pubchem.ncbi.nlm.nih.gov | |
Description | Data deposited in or computed by PubChem | |
CAS No. |
132172-61-3 | |
Record name | Dotap chloride | |
Source | ChemIDplus | |
URL | https://pubchem.ncbi.nlm.nih.gov/substance/?source=chemidplus&sourceid=0132172613 | |
Description | ChemIDplus is a free, web search system that provides access to the structure and nomenclature authority files used for the identification of chemical substances cited in National Library of Medicine (NLM) databases, including the TOXNET system. | |
Record name | DOTAP CHLORIDE | |
Source | FDA Global Substance Registration System (GSRS) | |
URL | https://gsrs.ncats.nih.gov/ginas/app/beta/substances/3R78UC794Z | |
Description | The FDA Global Substance Registration System (GSRS) enables the efficient and accurate exchange of information on what substances are in regulated products. Instead of relying on names, which vary across regulatory domains, countries, and regions, the GSRS knowledge base makes it possible for substances to be defined by standardized, scientific descriptions. | |
Explanation | Unless otherwise noted, the contents of the FDA website (www.fda.gov), both text and graphics, are not copyrighted. They are in the public domain and may be republished, reprinted and otherwise used freely by anyone without the need to obtain permission from FDA. Credit to the U.S. Food and Drug Administration as the source is appreciated but not required. | |
Retrosynthesis Analysis
AI-Powered Synthesis Planning: Our tool employs the Template_relevance Pistachio, Template_relevance Bkms_metabolic, Template_relevance Pistachio_ringbreaker, Template_relevance Reaxys, Template_relevance Reaxys_biocatalysis model, leveraging a vast database of chemical reactions to predict feasible synthetic routes.
One-Step Synthesis Focus: Specifically designed for one-step synthesis, it provides concise and direct routes for your target compounds, streamlining the synthesis process.
Accurate Predictions: Utilizing the extensive PISTACHIO, BKMS_METABOLIC, PISTACHIO_RINGBREAKER, REAXYS, REAXYS_BIOCATALYSIS database, our tool offers high-accuracy predictions, reflecting the latest in chemical research and data.
Strategy Settings
Precursor scoring | Relevance Heuristic |
---|---|
Min. plausibility | 0.01 |
Model | Template_relevance |
Template Set | Pistachio/Bkms_metabolic/Pistachio_ringbreaker/Reaxys/Reaxys_biocatalysis |
Top-N result to add to graph | 6 |
Feasible Synthetic Routes
Disclaimer and Information on In-Vitro Research Products
Please be aware that all articles and product information presented on BenchChem are intended solely for informational purposes. The products available for purchase on BenchChem are specifically designed for in-vitro studies, which are conducted outside of living organisms. In-vitro studies, derived from the Latin term "in glass," involve experiments performed in controlled laboratory settings using cells or tissues. It is important to note that these products are not categorized as medicines or drugs, and they have not received approval from the FDA for the prevention, treatment, or cure of any medical condition, ailment, or disease. We must emphasize that any form of bodily introduction of these products into humans or animals is strictly prohibited by law. It is essential to adhere to these guidelines to ensure compliance with legal and ethical standards in research and experimentation.