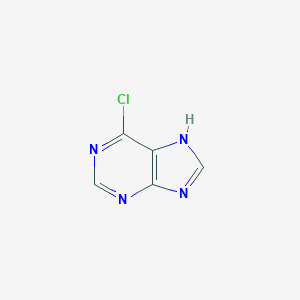
6-Chloropurine
Overview
Description
6-Chloropurine is a chemical compound with the molecular formula C5H3ClN4 and a molecular weight of 154.56 g/mol . It is a derivative of purine, a heterocyclic aromatic organic compound. This compound is known for its pale yellow crystalline appearance and is relatively stable under standard conditions .
Preparation Methods
Synthetic Routes and Reaction Conditions: 6-Chloropurine can be synthesized by reacting hypoxanthine with phosphoryl chloride in the presence of an unreactive base such as dimethylaniline . The reaction typically involves heating hypoxanthine under pressure with phosphoryl chloride and then adding water to the mixture .
Industrial Production Methods: In industrial settings, the preparation of this compound involves similar methods but on a larger scale. The reaction is carried out in a controlled environment to ensure the purity and yield of the product. The compound is then purified through crystallization from water .
Chemical Reactions Analysis
Types of Reactions: 6-Chloropurine undergoes various chemical reactions, including:
Nucleophilic Substitution: This is a common reaction where the chlorine atom is replaced by other nucleophiles.
Alkylation: It can be alkylated to form 9-alkylated adenines via the Mitsunobu reaction with various alcohols.
Catalytic Dehydrogenation: This reaction is used to prepare purine from this compound.
Common Reagents and Conditions:
Phosphoryl Chloride: Used in the initial synthesis of this compound from hypoxanthine.
Dimethylaniline: Acts as an unreactive base in the synthesis process.
Palladium Catalyst: Used in nucleophilic substitution reactions.
Major Products Formed:
Aryl or Alkyl Substituted Purines: Formed through nucleophilic substitution reactions.
9-Alkylated Adenines: Formed via the Mitsunobu reaction.
Scientific Research Applications
6-Chloropurine has a wide range of applications in scientific research:
Chemistry: It is used as a starting material for the synthesis of various purine derivatives.
Biology: It is used to study the kinetics and substrate specificity of enzymes such as adenosine deaminase.
Medicine: this compound and its derivatives have been investigated for their antileukemic properties.
Industry: It is used in the preparation of nucleoside derivatives and other chemical compounds.
Mechanism of Action
The mechanism of action of 6-Chloropurine involves its interaction with specific molecular targets. For instance, its riboside derivative, this compound riboside, is phosphorylated to form nucleoside monophosphates, which can inhibit enzymes such as inosine monophosphate dehydrogenase . This inhibition affects the synthesis of nucleotides, thereby exerting its biological effects.
Comparison with Similar Compounds
6-Mercaptopurine: Another purine derivative used in the treatment of leukemia.
6-Thioguanine: Used in the treatment of acute myeloid leukemia.
Adenine: A naturally occurring purine base found in DNA and RNA.
Comparison: 6-Chloropurine is unique due to its chlorine substitution at the 6-position, which allows for specific chemical reactions and applications that are not possible with other purine derivatives . For example, its ability to undergo nucleophilic substitution reactions makes it a valuable intermediate in the synthesis of various purine analogs .
Biological Activity
6-Chloropurine is a purine derivative that has garnered attention in medicinal chemistry due to its diverse biological activities. This article delves into the synthesis, biological properties, and potential applications of this compound, particularly focusing on its antifungal, antitumor, and antimicrobial effects.
Chemical Structure and Synthesis
This compound can be synthesized from commercially available 2-amino-6-chloropurine through various chemical reactions. The synthesis often involves the introduction of different substituents at the 6-position of the purine ring to enhance its biological activity. For example, novel derivatives have been synthesized using alkoxylation methods, which have shown promising antifungal properties against various strains.
Antifungal Activity
Recent studies have demonstrated that several derivatives of this compound exhibit significant antifungal activity. A study synthesized a series of 6-substituted purines, including derivatives such as 2-fluoro-6-chloropurine and 2-hydroxy-6-chloropurine. These compounds were tested against fungal strains such as Candida tropicalis, Aspergillus niger, and Bacillus subtilis. The results indicated that specific derivatives showed potent antifungal effects comparable to established antifungal agents like fluconazole.
Compound | Activity Against Candida tropicalis | Activity Against Aspergillus niger | Activity Against Bacillus subtilis |
---|---|---|---|
7 | Good | Moderate | Good |
8 | Good | Good | Good |
11 | Moderate | Good | Fairly good |
13 | Fairly good | Good | Good |
15 | Good | Moderate | Fairly good |
16 | Moderate | Fairly good | Good |
The study concluded that compounds such as 7, 8, 11, 13, 15, and 16 could serve as lead compounds for further development in antifungal therapies due to their effectiveness against multiple strains .
Antitumor Properties
In addition to antifungal properties, derivatives of this compound have shown potential as antitumor agents. The mechanism of action is believed to involve the inhibition of DNA synthesis in cancer cells. Research indicates that these compounds can induce apoptosis in various cancer cell lines by disrupting DNA replication processes.
A notable study highlighted the synthesis of O6-alkylguanine derivatives from 2-amino-6-chloropurine, which were screened for their cytotoxic effects on cancer cells. Some derivatives displayed significant cytotoxicity, suggesting their potential use in cancer treatment .
Case Studies and Research Findings
- Study on Antifungal Activity : A comprehensive evaluation of several new compounds derived from this compound revealed that certain derivatives exhibited enhanced activity against fungal pathogens. The study utilized standard antifungal testing protocols and reported that some compounds were more effective than existing treatments .
- Antitumor Screening : A series of experiments conducted on human cancer cell lines demonstrated that modifications at the N7 position of the purine ring significantly increased cytotoxicity. This finding suggests that targeted modifications can enhance the therapeutic potential of purine derivatives .
- Mechanistic Insights : Research into the mechanisms by which these compounds exert their biological effects has shown that they may interfere with nucleotide metabolism and DNA repair processes in cells. This interference can lead to increased mutations and cell death in rapidly dividing cells, such as those found in tumors .
Properties
IUPAC Name |
6-chloro-7H-purine | |
---|---|---|
Source | PubChem | |
URL | https://pubchem.ncbi.nlm.nih.gov | |
Description | Data deposited in or computed by PubChem | |
InChI |
InChI=1S/C5H3ClN4/c6-4-3-5(9-1-7-3)10-2-8-4/h1-2H,(H,7,8,9,10) | |
Source | PubChem | |
URL | https://pubchem.ncbi.nlm.nih.gov | |
Description | Data deposited in or computed by PubChem | |
InChI Key |
ZKBQDFAWXLTYKS-UHFFFAOYSA-N | |
Source | PubChem | |
URL | https://pubchem.ncbi.nlm.nih.gov | |
Description | Data deposited in or computed by PubChem | |
Canonical SMILES |
C1=NC2=C(N1)C(=NC=N2)Cl | |
Source | PubChem | |
URL | https://pubchem.ncbi.nlm.nih.gov | |
Description | Data deposited in or computed by PubChem | |
Molecular Formula |
C5H3ClN4 | |
Record name | 6-chloropurine | |
Source | Wikipedia | |
URL | https://en.wikipedia.org/wiki/Dictionary_of_chemical_formulas | |
Description | Chemical information link to Wikipedia. | |
Source | PubChem | |
URL | https://pubchem.ncbi.nlm.nih.gov | |
Description | Data deposited in or computed by PubChem | |
DSSTOX Substance ID |
DTXSID00861673 | |
Record name | 6-Chloropurine | |
Source | EPA DSSTox | |
URL | https://comptox.epa.gov/dashboard/DTXSID00861673 | |
Description | DSSTox provides a high quality public chemistry resource for supporting improved predictive toxicology. | |
Molecular Weight |
154.56 g/mol | |
Source | PubChem | |
URL | https://pubchem.ncbi.nlm.nih.gov | |
Description | Data deposited in or computed by PubChem | |
Physical Description |
Solid; [Merck Index] White powder; [Sigma-Aldrich MSDS] | |
Record name | 6-Chloropurine | |
Source | Haz-Map, Information on Hazardous Chemicals and Occupational Diseases | |
URL | https://haz-map.com/Agents/19828 | |
Description | Haz-Map® is an occupational health database designed for health and safety professionals and for consumers seeking information about the adverse effects of workplace exposures to chemical and biological agents. | |
Explanation | Copyright (c) 2022 Haz-Map(R). All rights reserved. Unless otherwise indicated, all materials from Haz-Map are copyrighted by Haz-Map(R). No part of these materials, either text or image may be used for any purpose other than for personal use. Therefore, reproduction, modification, storage in a retrieval system or retransmission, in any form or by any means, electronic, mechanical or otherwise, for reasons other than personal use, is strictly prohibited without prior written permission. | |
CAS No. |
87-42-3, 133762-83-1 | |
Record name | 6-Chloropurine | |
Source | CAS Common Chemistry | |
URL | https://commonchemistry.cas.org/detail?cas_rn=87-42-3 | |
Description | CAS Common Chemistry is an open community resource for accessing chemical information. Nearly 500,000 chemical substances from CAS REGISTRY cover areas of community interest, including common and frequently regulated chemicals, and those relevant to high school and undergraduate chemistry classes. This chemical information, curated by our expert scientists, is provided in alignment with our mission as a division of the American Chemical Society. | |
Explanation | The data from CAS Common Chemistry is provided under a CC-BY-NC 4.0 license, unless otherwise stated. | |
Record name | 6-Chloropurine | |
Source | ChemIDplus | |
URL | https://pubchem.ncbi.nlm.nih.gov/substance/?source=chemidplus&sourceid=0000087423 | |
Description | ChemIDplus is a free, web search system that provides access to the structure and nomenclature authority files used for the identification of chemical substances cited in National Library of Medicine (NLM) databases, including the TOXNET system. | |
Record name | 6-Chloropurin-9-yl | |
Source | ChemIDplus | |
URL | https://pubchem.ncbi.nlm.nih.gov/substance/?source=chemidplus&sourceid=0133762831 | |
Description | ChemIDplus is a free, web search system that provides access to the structure and nomenclature authority files used for the identification of chemical substances cited in National Library of Medicine (NLM) databases, including the TOXNET system. | |
Record name | 6-CHLOROPURINE | |
Source | DTP/NCI | |
URL | https://dtp.cancer.gov/dtpstandard/servlet/dwindex?searchtype=NSC&outputformat=html&searchlist=744 | |
Description | The NCI Development Therapeutics Program (DTP) provides services and resources to the academic and private-sector research communities worldwide to facilitate the discovery and development of new cancer therapeutic agents. | |
Explanation | Unless otherwise indicated, all text within NCI products is free of copyright and may be reused without our permission. Credit the National Cancer Institute as the source. | |
Record name | 6-Chloropurine | |
Source | EPA DSSTox | |
URL | https://comptox.epa.gov/dashboard/DTXSID00861673 | |
Description | DSSTox provides a high quality public chemistry resource for supporting improved predictive toxicology. | |
Record name | 6-chloropurine | |
Source | European Chemicals Agency (ECHA) | |
URL | https://echa.europa.eu/substance-information/-/substanceinfo/100.001.587 | |
Description | The European Chemicals Agency (ECHA) is an agency of the European Union which is the driving force among regulatory authorities in implementing the EU's groundbreaking chemicals legislation for the benefit of human health and the environment as well as for innovation and competitiveness. | |
Explanation | Use of the information, documents and data from the ECHA website is subject to the terms and conditions of this Legal Notice, and subject to other binding limitations provided for under applicable law, the information, documents and data made available on the ECHA website may be reproduced, distributed and/or used, totally or in part, for non-commercial purposes provided that ECHA is acknowledged as the source: "Source: European Chemicals Agency, http://echa.europa.eu/". Such acknowledgement must be included in each copy of the material. ECHA permits and encourages organisations and individuals to create links to the ECHA website under the following cumulative conditions: Links can only be made to webpages that provide a link to the Legal Notice page. | |
Record name | 6-CHLOROPURINE | |
Source | FDA Global Substance Registration System (GSRS) | |
URL | https://gsrs.ncats.nih.gov/ginas/app/beta/substances/OH8700156W | |
Description | The FDA Global Substance Registration System (GSRS) enables the efficient and accurate exchange of information on what substances are in regulated products. Instead of relying on names, which vary across regulatory domains, countries, and regions, the GSRS knowledge base makes it possible for substances to be defined by standardized, scientific descriptions. | |
Explanation | Unless otherwise noted, the contents of the FDA website (www.fda.gov), both text and graphics, are not copyrighted. They are in the public domain and may be republished, reprinted and otherwise used freely by anyone without the need to obtain permission from FDA. Credit to the U.S. Food and Drug Administration as the source is appreciated but not required. | |
Synthesis routes and methods I
Procedure details
Synthesis routes and methods II
Procedure details
Synthesis routes and methods III
Procedure details
Synthesis routes and methods IV
Procedure details
Synthesis routes and methods V
Procedure details
Retrosynthesis Analysis
AI-Powered Synthesis Planning: Our tool employs the Template_relevance Pistachio, Template_relevance Bkms_metabolic, Template_relevance Pistachio_ringbreaker, Template_relevance Reaxys, Template_relevance Reaxys_biocatalysis model, leveraging a vast database of chemical reactions to predict feasible synthetic routes.
One-Step Synthesis Focus: Specifically designed for one-step synthesis, it provides concise and direct routes for your target compounds, streamlining the synthesis process.
Accurate Predictions: Utilizing the extensive PISTACHIO, BKMS_METABOLIC, PISTACHIO_RINGBREAKER, REAXYS, REAXYS_BIOCATALYSIS database, our tool offers high-accuracy predictions, reflecting the latest in chemical research and data.
Strategy Settings
Precursor scoring | Relevance Heuristic |
---|---|
Min. plausibility | 0.01 |
Model | Template_relevance |
Template Set | Pistachio/Bkms_metabolic/Pistachio_ringbreaker/Reaxys/Reaxys_biocatalysis |
Top-N result to add to graph | 6 |
Feasible Synthetic Routes
A: 6-Chloropurine acts as a prodrug, meaning it is metabolized in the body into its active form. One of its primary mechanisms of action is conversion to 6-thioinosinic acid (6-thioIMP). [] 6-thioIMP then inhibits purine nucleotide biosynthesis, ultimately interfering with DNA and RNA synthesis. [, ] This inhibition preferentially targets rapidly dividing cells, such as cancer cells. [, ]
A:
- UV-Vis: λmax in methanol = 265 nm []
- 1H NMR (DMSO-d6): δ 8.74 (s, 1H, H-8), 8.23 (s, 1H, H-2) []
- 13C NMR (DMSO-d6): δ 152.2 (C-2), 151.8 (C-4), 151.1 (C-6), 142.1 (C-8), 131.2 (C-5) []
A: this compound exhibits varying solubility depending on the solvent. It demonstrates higher solubility in polar aprotic solvents like dimethyl sulfoxide (DMSO) and N,N-dimethylformamide (DMF) compared to water. [] This solubility difference is crucial for choosing appropriate solvents during chemical synthesis and formulation development.
A: While not typically used as a catalyst itself, this compound serves as a vital starting material for synthesizing various biologically active compounds. It acts as an electrophile in various nucleophilic aromatic substitution reactions, enabling the introduction of different substituents at the 6-position of the purine ring. [, , , , ] This versatility makes it valuable in medicinal chemistry for developing novel therapeutics.
ANone: Modifications to the this compound structure greatly influence its biological activity:
- Substitution at the 6-position: Replacing chlorine with other groups like thiol (-SH), alkylthio (-SR), or amino (-NH2) significantly alters its pharmacological profile. [, , , ] These modifications can impact its metabolism, target affinity, and overall efficacy.
- N9-Alkylation: Introducing alkyl groups at the N9 position of the purine ring can impact its antitumor and antiviral activity. [, ] The size and nature of the alkyl group can influence its interaction with enzymes and cellular targets.
- Ribosylation: Attaching a ribose sugar to the N9 position yields this compound riboside, affecting its cellular uptake and metabolism. [] This modification can influence its conversion to active metabolites and subsequent biological activity.
A: this compound is rapidly absorbed after administration and undergoes extensive metabolism. [] The liver plays a major role in its metabolism, leading to the formation of various metabolites, including 6-chlorouric acid and 6-thioinosinic acid. [, , ] Excretion occurs primarily through the urine, with a small portion eliminated in feces. []
A:
- In Vitro: this compound has shown efficacy against various cancer cell lines, including murine leukemias (L1210 and P388) and human breast carcinoma (MCF7). []
- In Vivo: In animal models, this compound demonstrated activity against Sarcoma 180 ascites cells, inhibiting tumor growth. []
ANone: Resistance to this compound can arise from various mechanisms, including:
- Decreased uptake or increased efflux: Changes in cellular transport can limit drug accumulation within the cells, reducing its effectiveness. []
- Altered metabolism: Mutations in enzymes responsible for this compound metabolism, such as hypoxanthine-guanine phosphoribosyltransferase (HGPRT), can lead to resistance. []
A: this compound can cause a range of side effects, with myelosuppression (suppression of bone marrow activity) being the most common dose-limiting toxicity. [, ] Gastrointestinal disturbances, hepatotoxicity (liver damage), and an increased risk of infection are also possible adverse effects. [, ]
Disclaimer and Information on In-Vitro Research Products
Please be aware that all articles and product information presented on BenchChem are intended solely for informational purposes. The products available for purchase on BenchChem are specifically designed for in-vitro studies, which are conducted outside of living organisms. In-vitro studies, derived from the Latin term "in glass," involve experiments performed in controlled laboratory settings using cells or tissues. It is important to note that these products are not categorized as medicines or drugs, and they have not received approval from the FDA for the prevention, treatment, or cure of any medical condition, ailment, or disease. We must emphasize that any form of bodily introduction of these products into humans or animals is strictly prohibited by law. It is essential to adhere to these guidelines to ensure compliance with legal and ethical standards in research and experimentation.