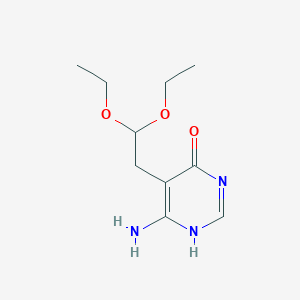
6-Amino-5-(2,2-diethoxyethyl)pyrimidin-4-ol
Overview
Description
6-Amino-5-(2,2-diethoxyethyl)pyrimidin-4-ol is a chemical compound with the molecular formula C10H17N3O3 and a molecular weight of 227.26 g/mol . It is a pyrimidine derivative, characterized by the presence of an amino group at the 6th position and a diethoxyethyl group at the 5th position of the pyrimidine ring
Preparation Methods
Synthetic Routes and Reaction Conditions
The synthesis of 6-Amino-5-(2,2-diethoxyethyl)pyrimidin-4-ol can be achieved through multiple synthetic routes. One common method involves the reaction of 2-cyano-4,4-diethoxybutanoic acid ethyl ester with guanidine hydrochloride . The reaction typically proceeds under reflux conditions in the presence of a suitable solvent, such as ethanol, and yields the desired product with a moderate yield of approximately 66% .
Industrial Production Methods
Industrial production methods for this compound are not well-documented in the literature. the synthesis methods used in laboratory settings can be scaled up for industrial production with appropriate optimization of reaction conditions and purification processes.
Chemical Reactions Analysis
Types of Reactions
6-Amino-5-(2,2-diethoxyethyl)pyrimidin-4-ol undergoes various types of chemical reactions, including:
Oxidation: The compound can be oxidized under specific conditions to form corresponding oxidized derivatives.
Reduction: Reduction reactions can be performed to modify the functional groups present in the compound.
Substitution: The amino group and the diethoxyethyl group can participate in substitution reactions with suitable reagents.
Common Reagents and Conditions
Common reagents used in the reactions of this compound include oxidizing agents like hydrogen peroxide, reducing agents such as sodium borohydride, and various nucleophiles for substitution reactions . The reaction conditions vary depending on the desired transformation, but typical conditions include controlled temperatures and the use of appropriate solvents.
Major Products
The major products formed from the reactions of this compound depend on the specific reaction being performed. For example, oxidation reactions may yield oxidized pyrimidine derivatives, while substitution reactions can result in the formation of various substituted pyrimidines .
Scientific Research Applications
6-Amino-5-(2,2-diethoxyethyl)pyrimidin-4-ol has several scientific research applications, including:
Mechanism of Action
The mechanism of action of 6-Amino-5-(2,2-diethoxyethyl)pyrimidin-4-ol is not fully understood. it is believed to interact with specific molecular targets and pathways in biological systems. The amino and diethoxyethyl groups may play a role in its binding affinity and activity towards these targets . Further research is needed to elucidate the exact molecular mechanisms and pathways involved.
Comparison with Similar Compounds
6-Amino-5-(2,2-diethoxyethyl)pyrimidin-4-ol can be compared with other similar pyrimidine derivatives, such as:
- 6-Amino-5-(2,2-diethoxyethyl)-4-hydroxy-2-mercaptopyrimidine
- 4-Hydroxy-6-methylpyrimidine
- 2-Chloropyrimidin-4-ol
These compounds share structural similarities but differ in their functional groups and chemical properties.
Properties
IUPAC Name |
4-amino-5-(2,2-diethoxyethyl)-1H-pyrimidin-6-one | |
---|---|---|
Source | PubChem | |
URL | https://pubchem.ncbi.nlm.nih.gov | |
Description | Data deposited in or computed by PubChem | |
InChI |
InChI=1S/C10H17N3O3/c1-3-15-8(16-4-2)5-7-9(11)12-6-13-10(7)14/h6,8H,3-5H2,1-2H3,(H3,11,12,13,14) | |
Source | PubChem | |
URL | https://pubchem.ncbi.nlm.nih.gov | |
Description | Data deposited in or computed by PubChem | |
InChI Key |
DLABUHZHXFNKMQ-UHFFFAOYSA-N | |
Source | PubChem | |
URL | https://pubchem.ncbi.nlm.nih.gov | |
Description | Data deposited in or computed by PubChem | |
Canonical SMILES |
CCOC(CC1=C(N=CNC1=O)N)OCC | |
Source | PubChem | |
URL | https://pubchem.ncbi.nlm.nih.gov | |
Description | Data deposited in or computed by PubChem | |
Molecular Formula |
C10H17N3O3 | |
Source | PubChem | |
URL | https://pubchem.ncbi.nlm.nih.gov | |
Description | Data deposited in or computed by PubChem | |
DSSTOX Substance ID |
DTXSID00289111 | |
Record name | 6-amino-5-(2,2-diethoxyethyl)pyrimidin-4-ol | |
Source | EPA DSSTox | |
URL | https://comptox.epa.gov/dashboard/DTXSID00289111 | |
Description | DSSTox provides a high quality public chemistry resource for supporting improved predictive toxicology. | |
Molecular Weight |
227.26 g/mol | |
Source | PubChem | |
URL | https://pubchem.ncbi.nlm.nih.gov | |
Description | Data deposited in or computed by PubChem | |
CAS No. |
7400-06-8 | |
Record name | 7400-06-8 | |
Source | DTP/NCI | |
URL | https://dtp.cancer.gov/dtpstandard/servlet/dwindex?searchtype=NSC&outputformat=html&searchlist=59250 | |
Description | The NCI Development Therapeutics Program (DTP) provides services and resources to the academic and private-sector research communities worldwide to facilitate the discovery and development of new cancer therapeutic agents. | |
Explanation | Unless otherwise indicated, all text within NCI products is free of copyright and may be reused without our permission. Credit the National Cancer Institute as the source. | |
Record name | 6-amino-5-(2,2-diethoxyethyl)pyrimidin-4-ol | |
Source | EPA DSSTox | |
URL | https://comptox.epa.gov/dashboard/DTXSID00289111 | |
Description | DSSTox provides a high quality public chemistry resource for supporting improved predictive toxicology. | |
Retrosynthesis Analysis
AI-Powered Synthesis Planning: Our tool employs the Template_relevance Pistachio, Template_relevance Bkms_metabolic, Template_relevance Pistachio_ringbreaker, Template_relevance Reaxys, Template_relevance Reaxys_biocatalysis model, leveraging a vast database of chemical reactions to predict feasible synthetic routes.
One-Step Synthesis Focus: Specifically designed for one-step synthesis, it provides concise and direct routes for your target compounds, streamlining the synthesis process.
Accurate Predictions: Utilizing the extensive PISTACHIO, BKMS_METABOLIC, PISTACHIO_RINGBREAKER, REAXYS, REAXYS_BIOCATALYSIS database, our tool offers high-accuracy predictions, reflecting the latest in chemical research and data.
Strategy Settings
Precursor scoring | Relevance Heuristic |
---|---|
Min. plausibility | 0.01 |
Model | Template_relevance |
Template Set | Pistachio/Bkms_metabolic/Pistachio_ringbreaker/Reaxys/Reaxys_biocatalysis |
Top-N result to add to graph | 6 |
Feasible Synthetic Routes
Disclaimer and Information on In-Vitro Research Products
Please be aware that all articles and product information presented on BenchChem are intended solely for informational purposes. The products available for purchase on BenchChem are specifically designed for in-vitro studies, which are conducted outside of living organisms. In-vitro studies, derived from the Latin term "in glass," involve experiments performed in controlled laboratory settings using cells or tissues. It is important to note that these products are not categorized as medicines or drugs, and they have not received approval from the FDA for the prevention, treatment, or cure of any medical condition, ailment, or disease. We must emphasize that any form of bodily introduction of these products into humans or animals is strictly prohibited by law. It is essential to adhere to these guidelines to ensure compliance with legal and ethical standards in research and experimentation.