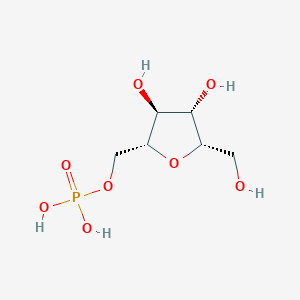
2,5-Anhydro-D-glucitol-6-phosphate
Overview
Description
2,5-Anhydro-D-glucitol-6-phosphate is a phosphorylated derivative of D-glucitol 6 phosphate. It is a major metabolic intermediate in the glycolytic pathway, produced by the enzyme phosphofructokinase and hydrolyzed by the enzyme pyruvate kinase . It is a colorless or white crystalline solid, soluble in water but not in organic solvents .
Synthesis Analysis
The synthesis of 2,5-Anhydro-D-glucitol-6-phosphate is complex and generally involves multiple steps. One method uses D-glucose as the starting material, which undergoes enzymatic catalysis and chemical reactions, through the generation and transformation of intermediates, to finally obtain the target product .Molecular Structure Analysis
The molecular formula of 2,5-Anhydro-D-glucitol-6-phosphate is C6H13O8P . It contains a total of 28 bonds, including 15 non-H bonds, 1 multiple bond, 4 rotatable bonds, 1 double bond, 1 five-membered ring, 5 hydroxyl groups, 1 primary alcohol, 2 secondary alcohols, 1 ether (aliphatic), 1 phosphate/thiophosphate, and 1 Oxolane .Chemical Reactions Analysis
2,5-Anhydro-D-glucitol-6-phosphate has significant applications in the biochemical and pharmaceutical fields. It can be used as a substrate for studying glycolysis and sugar metabolism, which is crucial for understanding cellular metabolic processes. Additionally, it is used as a substrate for some enzymes to detect and measure enzyme activity .Physical And Chemical Properties Analysis
2,5-Anhydro-D-glucitol-6-phosphate has a molecular weight of 244.14 g/mol . It has a density of 1.86 g/cm³ and a melting point of approximately 215-225°C .Scientific Research Applications
Cancer Research
2,5-Anhydro-D-glucitol-6-phosphate is a powerful research component in the search for therapeutic measures to regulate glycolysis and eventually, cancer . It acts as an effective competitive inhibitor of phosphofructokinase-1 (PFK-1), a rate-determining enzyme of glycolysis . This enzyme regulates the oxidation of glucose in cellular respiration .
Diabetes Research
2,5-Anhydro-D-glucitol-6-phosphate is known for its potential therapeutic applications in research of diabetes and related metabolic disorders . It could be a tool in the development of novel drugs for diabetes management .
Biochemical and Physiological Research
Biochemicals that inhibit PFK, like 2,5-Anhydro-D-glucitol-6-phosphate, have many applications in biochemical and physiological research . They help in understanding the conversion of fructose 6-phosphate and ATP into fructose 1,6-bisphosphate (through PFK-1), fructose 2,6-bisphosphate (through PFK-2) and ADP .
Glycolysis and Sugar Metabolism Research
2,5-Anhydro-D-glucitol-6-phosphate can be used as a substrate for research into glycolysis and sugar metabolism . It plays a significant role in understanding cellular metabolic processes .
Enzyme Activity Research
2,5-Anhydro-D-glucitol-6-phosphate is also used as a substrate for some enzymes, used for detecting and measuring enzyme activity . This helps in understanding the function and regulation of these enzymes .
Drug Development
Given its role as an effective competitive inhibitor of PFK-1, 2,5-Anhydro-D-glucitol-6-phosphate can be used in the development of drugs that target this enzyme . This could lead to new treatments for conditions where PFK-1 plays a key role .
Mechanism of Action
Target of Action
The primary target of 2,5-Anhydro-D-glucitol-6-phosphate is the enzyme Pyruvate Kinase . This enzyme plays a crucial role in the glycolytic pathway, where it catalyzes the transfer of a phosphate group from phosphoenolpyruvate (PEP) to ADP, yielding one molecule of pyruvate and one molecule of ATP .
Mode of Action
2,5-Anhydro-D-glucitol-6-phosphate interacts with its target, Pyruvate Kinase, by acting as a limited stimulator . It is used as an analogue of the a-form, 2,5-anhydro-D-mannitol 1,6-bisphosphate, which is an excellent allosteric activator of Pyruvate Kinase .
Biochemical Pathways
2,5-Anhydro-D-glucitol-6-phosphate is a major metabolic intermediate in the glycolytic pathway . It is produced by the enzyme phosphofructokinase and hydrolyzed by the enzyme pyruvate kinase . This indicates that it plays a significant role in glucose metabolism and energy production within cells.
Pharmacokinetics
It is known to be soluble in water , which could potentially influence its absorption and distribution within the body
Result of Action
The interaction of 2,5-Anhydro-D-glucitol-6-phosphate with Pyruvate Kinase can influence the rate of glycolysis, thereby affecting glucose metabolism within cells . This could potentially have a range of downstream effects at the molecular and cellular levels, depending on the specific context and conditions.
properties
IUPAC Name |
[(2R,3S,4S,5S)-3,4-dihydroxy-5-(hydroxymethyl)oxolan-2-yl]methyl dihydrogen phosphate | |
---|---|---|
Source | PubChem | |
URL | https://pubchem.ncbi.nlm.nih.gov | |
Description | Data deposited in or computed by PubChem | |
InChI |
InChI=1S/C6H13O8P/c7-1-3-5(8)6(9)4(14-3)2-13-15(10,11)12/h3-9H,1-2H2,(H2,10,11,12)/t3-,4+,5+,6+/m0/s1 | |
Source | PubChem | |
URL | https://pubchem.ncbi.nlm.nih.gov | |
Description | Data deposited in or computed by PubChem | |
InChI Key |
XYTKBMAMGDBXPU-SLPGGIOYSA-N | |
Source | PubChem | |
URL | https://pubchem.ncbi.nlm.nih.gov | |
Description | Data deposited in or computed by PubChem | |
Canonical SMILES |
C(C1C(C(C(O1)COP(=O)(O)O)O)O)O | |
Source | PubChem | |
URL | https://pubchem.ncbi.nlm.nih.gov | |
Description | Data deposited in or computed by PubChem | |
Isomeric SMILES |
C([C@H]1[C@H]([C@@H]([C@H](O1)COP(=O)(O)O)O)O)O | |
Source | PubChem | |
URL | https://pubchem.ncbi.nlm.nih.gov | |
Description | Data deposited in or computed by PubChem | |
Molecular Formula |
C6H13O8P | |
Source | PubChem | |
URL | https://pubchem.ncbi.nlm.nih.gov | |
Description | Data deposited in or computed by PubChem | |
Molecular Weight |
244.14 g/mol | |
Source | PubChem | |
URL | https://pubchem.ncbi.nlm.nih.gov | |
Description | Data deposited in or computed by PubChem | |
Product Name |
2,5-Anhydro-D-glucitol-6-phosphate |
Retrosynthesis Analysis
AI-Powered Synthesis Planning: Our tool employs the Template_relevance Pistachio, Template_relevance Bkms_metabolic, Template_relevance Pistachio_ringbreaker, Template_relevance Reaxys, Template_relevance Reaxys_biocatalysis model, leveraging a vast database of chemical reactions to predict feasible synthetic routes.
One-Step Synthesis Focus: Specifically designed for one-step synthesis, it provides concise and direct routes for your target compounds, streamlining the synthesis process.
Accurate Predictions: Utilizing the extensive PISTACHIO, BKMS_METABOLIC, PISTACHIO_RINGBREAKER, REAXYS, REAXYS_BIOCATALYSIS database, our tool offers high-accuracy predictions, reflecting the latest in chemical research and data.
Strategy Settings
Precursor scoring | Relevance Heuristic |
---|---|
Min. plausibility | 0.01 |
Model | Template_relevance |
Template Set | Pistachio/Bkms_metabolic/Pistachio_ringbreaker/Reaxys/Reaxys_biocatalysis |
Top-N result to add to graph | 6 |
Feasible Synthetic Routes
Q & A
Q1: How does 2,5-Anhydro-D-glucitol-6-phosphate interact with phosphofructokinase (PFK), and what are the downstream effects?
A1: 2,5-Anhydro-D-glucitol-6-phosphate acts as a competitive inhibitor of PFK, specifically targeting the enzyme's fructose 6-phosphate (F6P) binding site. [] This binding prevents the natural substrate, F6P, from binding to PFK. As PFK is a key regulatory enzyme in glycolysis, inhibiting its activity leads to a decrease in glycolytic flux. [] This metabolic disruption can subsequently impact cancer cell growth and invasion, as demonstrated in bladder cancer models. []
Q2: What is known about the structure-activity relationship (SAR) of 2,5-Anhydro-D-glucitol-6-phosphate and its analogues in relation to PFK inhibition?
A2: Research indicates that the F6P binding site on PFK displays specific structural requirements for substrate and inhibitor binding. [] For instance, the presence and stereochemistry of hydroxyl groups on the sugar ring significantly impact binding affinity. 2,5-Anhydro-D-mannitol 1-phosphate, another analogue, also binds to the F6P site, highlighting the importance of the C-2 hydroxyl group for interaction. [] Further exploration of structural modifications could provide valuable insights for designing more potent and selective PFK inhibitors.
Disclaimer and Information on In-Vitro Research Products
Please be aware that all articles and product information presented on BenchChem are intended solely for informational purposes. The products available for purchase on BenchChem are specifically designed for in-vitro studies, which are conducted outside of living organisms. In-vitro studies, derived from the Latin term "in glass," involve experiments performed in controlled laboratory settings using cells or tissues. It is important to note that these products are not categorized as medicines or drugs, and they have not received approval from the FDA for the prevention, treatment, or cure of any medical condition, ailment, or disease. We must emphasize that any form of bodily introduction of these products into humans or animals is strictly prohibited by law. It is essential to adhere to these guidelines to ensure compliance with legal and ethical standards in research and experimentation.