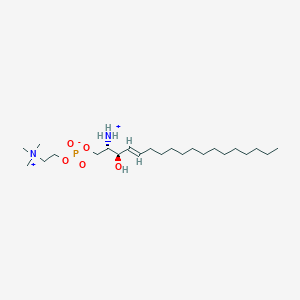
Sphingosylphosphorylcholine
Overview
Description
Sphingosylphosphorylcholine (SPC) is a bioactive sphingolipid derived from the hydrolysis of sphingomyelin. Structurally, it consists of a sphingosine backbone (a long-chain amino alcohol) linked to a phosphorylcholine group. Its molecular formula is C₂₃H₄₉N₂O₅P, with a molecular weight of 464.62 g/mol . SPC acts as a potent signaling molecule, engaging G protein-coupled receptors (GPCRs) such as OGR1 and GPR4 to regulate intracellular calcium mobilization, extracellular signal-regulated kinase (ERK) activation, and cellular processes like migration, proliferation, and apoptosis . Elevated SPC levels are implicated in metabolic syndrome and subarachnoid hemorrhage-induced vasospasm , while its neurotrophic properties are observed in cerebellar granule neurons .
Preparation Methods
Synthetic Routes and Reaction Conditions
Sphingosylphosphorylcholine can be synthesized through the hydrolysis of sphingomyelin by sphingomyelinase or sphingomyelin deacylase . The reaction conditions typically involve the use of specific enzymes that catalyze the hydrolysis process. The production of this compound from sphingomyelin is a precisely regulated enzymatic process .
Industrial Production Methods
Industrial production of this compound involves the extraction and purification of the compound from biological sources such as blood plasma or platelets . The process includes the use of chromatographic techniques to isolate and purify this compound from other sphingolipids and lipoproteins .
Chemical Reactions Analysis
Types of Reactions
Sphingosylphosphorylcholine undergoes various chemical reactions, including:
Oxidation: this compound can be oxidized to form different metabolites.
Reduction: Reduction reactions can modify the functional groups of this compound.
Substitution: This compound can participate in substitution reactions where functional groups are replaced by other groups.
Common Reagents and Conditions
Common reagents used in the reactions of this compound include oxidizing agents, reducing agents, and catalysts that facilitate substitution reactions . The reaction conditions vary depending on the desired product and the specific reaction being carried out.
Major Products Formed
The major products formed from the reactions of this compound include various metabolites that play significant roles in cellular processes . These products can influence cell signaling pathways and physiological functions.
Scientific Research Applications
Sphingosylphosphorylcholine has a wide range of scientific research applications, including:
Chemistry: this compound is studied for its chemical properties and reactions.
Biology: It plays a role in cell differentiation, proliferation, and apoptosis.
Medicine: This compound is involved in the modulation of cardiovascular functions and diseases.
Industry: This compound is used in the development of pharmaceuticals and therapeutic agents.
Mechanism of Action
Sphingosylphosphorylcholine exerts its effects through various molecular targets and pathways. It can act as a first messenger through G protein-coupled receptors or membrane lipid rafts, or as a second messenger mediating intracellular calcium release . The compound influences the functions of myocytes, vein endothelial cells, and vascular smooth muscle cells, thereby modulating cardiovascular functions . Additionally, this compound can activate pathways such as the Parkin and nuclear receptor subfamily group A member 2/optineurin pathways, leading to increased levels of mitophagy in affected myocardium .
Comparison with Similar Compounds
Comparison with Similar Compounds
Structural and Functional Comparison
Table 1: Structural and Receptor Affinity Profiles
Key Structural Differences:
- SPC vs. LPC : SPC has a sphingosine backbone, whereas LPC contains a glycerol backbone esterified with a single fatty acid. This structural divergence leads to distinct receptor affinities (e.g., GPR4 binds SPC with 4.4-fold higher affinity than LPC) .
- SPC vs. S1P : S1P replaces SPC’s phosphorylcholine group with a phosphate, enabling selective binding to EDG-family receptors (e.g., EDG1) .
Receptor Specificity and Signaling Pathways
Table 2: Receptor-Mediated Signaling Outcomes
- SPC vs. LPC : Both activate GPR4, but SPC’s higher affinity drives stronger ERK activation and DNA synthesis in Swiss 3T3 cells .
- SPC vs. S1P: SPC-induced vasoconstriction involves protein kinase C (PKC)-δ and non-store-operated Ca²⁺ entry, whereas S1P primarily activates store-operated channels .
Enzymatic Metabolism and Degradation
- NPP6: Hydrolyzes SPC and LPC to phosphorylcholine and monoacylglycerol, respectively. Prefers polyunsaturated LPC (18:2, 20:4) and SPC .
- Sphingomyelinases : Generate SPC from sphingomyelin, unlike phospholipases that produce LPC from phosphatidylcholine .
Table 3: Metabolic Pathways
Enzyme | Substrates | Products | Tissue Localization |
---|---|---|---|
NPP6 | SPC, LPC, glycerophosphorylcholine | Phosphorylcholine, MAG | Kidney, brain, heart |
Autotaxin | LPC | LPA | Ubiquitous |
Disease Associations
Biological Activity
Sphingosylphosphorylcholine (SPC) is a unique lysosphingolipid that exhibits a wide range of biological activities, influencing various physiological and pathological processes. This article delves into the mechanisms of action, effects on different cell types, and its implications in diseases, particularly cancer and inflammation.
SPC is derived from sphingomyelin through the action of sphingomyelin deacylase. It is present in blood and has been associated with several diseases, including cancer, atopic dermatitis, and Niemann-Pick disease (NPD) . Elevated levels of SPC have been observed in various pathological conditions, indicating its role as a potential biomarker and therapeutic target.
SPC exerts its biological effects primarily through interaction with specific receptors and modulation of intracellular signaling pathways:
- Receptor Activation : SPC can activate sphingosine-1-phosphate (S1P) receptors (S1P1-S1P5), leading to various cellular responses such as proliferation, migration, and differentiation .
- Calcium Mobilization : SPC has been shown to induce calcium release from the endoplasmic reticulum, resulting in acute elevations in cytosolic calcium levels. This effect is critical for various cellular functions, including muscle contraction and neurotransmitter release .
3. Biological Activities
The biological activities of SPC can be categorized based on its effects on different cell types:
3.1 Cancer Cells
SPC has been extensively studied for its role in cancer biology:
- Inhibition of Cell Growth : In neuroblastoma cell lines (NS-20Y, Neuro2a, N1E-115), SPC inhibited growth at concentrations below 150 µM while promoting neurite outgrowth .
- Impact on Tumor Microenvironment : SPC influences the tumor microenvironment by modulating immune cell functions and promoting angiogenesis .
Cell Type | Concentration | Effect | Mechanism |
---|---|---|---|
Mouse Neuroblastoma Cells | < 150 µM | Growth inhibition; neurite outgrowth | Sphingomyelin metabolism |
Human Bladder Carcinoma Cells | 10 µM | Migration and invasion decrease | GPCR signaling |
Anaplastic Thyroid Carcinoma | 1-10 µM | Proliferation and migration decrease | PI3K-Akt pathway |
3.2 Immune System
SPC plays a significant role in modulating immune responses:
- Inhibition of Plasma Cell Differentiation : Recent studies indicate that SPC inhibits the differentiation of B cells into plasma cells, potentially influencing autoimmune conditions such as multiple sclerosis .
- Regulation of Inflammatory Responses : Elevated levels of SPC are linked to increased inflammatory responses in conditions like atopic dermatitis .
4. Case Studies
Several case studies highlight the relevance of SPC in clinical settings:
- Atopic Dermatitis : A study found that SPC levels were significantly elevated in the stratum corneum of patients with atopic dermatitis compared to healthy controls. This increase correlated with decreased ceramide levels, suggesting a role for SPC in skin barrier dysfunction .
- Cancer Progression : In ovarian cancer patients, higher levels of SPC were detected in ascitic fluid, indicating its potential as a marker for disease progression .
5. Conclusion
This compound is a multifaceted lipid mediator with significant implications for various biological processes. Its ability to modulate cell proliferation, migration, and differentiation makes it a crucial player in both normal physiology and pathological conditions. Ongoing research into its mechanisms may pave the way for novel therapeutic strategies targeting SPC-related pathways.
Q & A
Basic Research Questions
Q. What methodologies are recommended for quantifying Sphingosylphosphorylcholine (SPC) levels in biological samples?
SPC quantification typically employs fluorometric or colorimetric assays using enzymatic hydrolysis. For example, the Phosphatidylcholine Assay Kit (modified for SPC detection) involves:
- Sample Preparation : Cells (2 × 10⁶ cells) or tissues (10 mg) are homogenized in cold assay buffer, centrifuged to remove debris, and supernatants are analyzed .
- Reagent Handling : Enzymes (e.g., PC Hydrolysis Enzyme) and standards are reconstituted in assay buffer, aliquoted, and stored at -20°C to avoid freeze-thaw degradation .
- Assay Procedure : Samples (1–50 µL) are mixed with Reaction Mix (50 µL/well), incubated for 30 minutes at RT, and measured via microplate reader (OD570 nm for colorimetric; Ex/Em 535/587 nm for fluorometric) .
- Data Normalization : Background subtraction and duplicate averaging are critical. Samples exceeding standard ranges require dilution and reanalysis .
Q. How does SPC influence intracellular calcium signaling in neuronal models?
SPC modulates calcium release through ryanodine receptors (RyRs) in neurons. In cerebellar granule neurons (CGNs), SPC activates RyRs via calmodulin-dependent and -independent pathways , altering single-channel gating properties and promoting calcium flux. This mechanism is concentration-dependent, with thresholds at 1–10 µM . Experimental validation requires calcium imaging (e.g., Fura-2 AM) paired with RyR inhibitors (e.g., dantrolene) to isolate SPC-specific effects .
Advanced Research Questions
Q. How can researchers resolve contradictions in SPC receptor specificity (e.g., GPR4 vs. OGR1)?
Conflicting reports on SPC’s receptor targets (e.g., retracted claims about GPR4 and ovarian cancer GPCRs ) highlight the need for rigorous validation:
- Pharmacological Profiling : Use receptor-specific antagonists (e.g., SEW2871 for EDG-1) and siRNA knockdowns to confirm SPC-GPCR interactions .
- Biochemical Assays : Measure downstream signaling (e.g., IP3 accumulation, ERK phosphorylation) in receptor-deficient cell lines .
- Structural Studies : Molecular docking simulations predict SPC-receptor binding affinities, which can be validated via surface plasmon resonance (SPR) .
Q. What experimental designs address variability in SPC’s role in metabolic syndrome?
SPC plasma levels are elevated in metabolic syndrome patients (e.g., +30% vs. controls), correlating with BMI and inflammatory markers like sCD163 . To dissect causality:
- Longitudinal Cohort Studies : Track SPC levels in pre-diabetic vs. metabolic syndrome cohorts using LC-MS/MS for precise lipidomics .
- Mechanistic Models : Use adipose-specific Sphk1 knockout mice to assess SPC’s role in adipokine secretion and insulin resistance .
- Intervention Trials : Test SPC-lowering agents (e.g., myriocin) on inflammatory endpoints (e.g., IL-6, TNF-α) in patient-derived adipocytes .
Q. How does SPC regulate smooth muscle differentiation in stem cell therapies?
SPC induces smooth muscle-like differentiation in human mesenchymal stem cells (hMSCs) via TGF-β/Smad3-dependent pathways :
- Protocol : Treat hMSCs with 5–20 µM SPC for 7 days. Confirm differentiation via α-SMA immunofluorescence and contractility assays .
- Pathway Inhibition : Co-treatment with Smad3 inhibitors (e.g., SIS3) or TGF-β neutralizing antibodies blocks SPC effects, confirming pathway specificity .
- Functional Outcomes : Engineered tissues (e.g., vascular grafts) are tested for mechanical strength and calcium sensitivity .
Q. What are the best practices for mitigating interference in SPC assays (e.g., choline contamination)?
Choline in samples can inflate SPC readings via enzymatic cross-reactivity. Solutions include:
- Background Controls : Dedicate wells to sample + assay buffer without enzymes to quantify choline-driven signals .
- Pre-Treatment : Incubate samples with choline oxidase to degrade free choline prior to SPC measurement .
- Chromatographic Separation : Use HPLC-MS to distinguish SPC from phosphatidylcholine and lysophosphatidylcholine .
Q. How do SPC’s neurotrophic effects vary between neuronal subtypes?
SPC promotes survival in cerebellar granule neurons (CGNs) but induces apoptosis in PC12 cells under serum-free conditions:
- CGNs : SPC (10 µM) upregulates BDNF and TrkB via PI3K/Akt, validated via phospho-Akt Western blots .
- PC12 Cells : SPC (≥20 µM) activates JNK/c-Jun, leading to caspase-3 cleavage. Co-treatment with JNK inhibitors (SP600125) rescues viability .
- Experimental Controls : Include serum-starved vs. NGF-treated cells to isolate SPC-specific neurotrophic/apoptotic pathways .
Q. Data Contradiction Analysis
Q. Why do studies report conflicting roles for SPC in cancer progression?
SPC exhibits dual pro-/anti-tumor effects depending on context:
- Pro-Tumor : In ovarian cancer, SPC (via EMP2 ubiquitination) stabilizes oncogenic kinases (e.g., FAK), enhancing invasion .
- Anti-Tumor : In breast cancer, SPC downregulates MMP-9 via p38 MAPK, reducing metastasis .
Resolution Strategies : - Use isogenic cell lines with EMP2 knockout to isolate tumor-specific mechanisms .
- Compare SPC’s dose-response curves (0.1–50 µM) across cancer subtypes .
Properties
IUPAC Name |
(2-amino-3-hydroxyoctadec-4-enyl) 2-(trimethylazaniumyl)ethyl phosphate | |
---|---|---|
Details | Computed by LexiChem 2.6.6 (PubChem release 2019.06.18) | |
Source | PubChem | |
URL | https://pubchem.ncbi.nlm.nih.gov | |
Description | Data deposited in or computed by PubChem | |
InChI |
InChI=1S/C23H49N2O5P/c1-5-6-7-8-9-10-11-12-13-14-15-16-17-18-23(26)22(24)21-30-31(27,28)29-20-19-25(2,3)4/h17-18,22-23,26H,5-16,19-21,24H2,1-4H3 | |
Details | Computed by InChI 1.0.5 (PubChem release 2019.06.18) | |
Source | PubChem | |
URL | https://pubchem.ncbi.nlm.nih.gov | |
Description | Data deposited in or computed by PubChem | |
InChI Key |
JLVSPVFPBBFMBE-UHFFFAOYSA-N | |
Details | Computed by InChI 1.0.5 (PubChem release 2019.06.18) | |
Source | PubChem | |
URL | https://pubchem.ncbi.nlm.nih.gov | |
Description | Data deposited in or computed by PubChem | |
Canonical SMILES |
CCCCCCCCCCCCCC=CC(C(COP(=O)([O-])OCC[N+](C)(C)C)N)O | |
Details | Computed by OEChem 2.1.5 (PubChem release 2019.06.18) | |
Source | PubChem | |
URL | https://pubchem.ncbi.nlm.nih.gov | |
Description | Data deposited in or computed by PubChem | |
Molecular Formula |
C23H49N2O5P | |
Details | Computed by PubChem 2.1 (PubChem release 2019.06.18) | |
Source | PubChem | |
URL | https://pubchem.ncbi.nlm.nih.gov | |
Description | Data deposited in or computed by PubChem | |
DSSTOX Substance ID |
DTXSID40274460 | |
Record name | 2-Amino-3-hydroxyoctadec-4-en-1-yl 2-(trimethylazaniumyl)ethyl phosphate | |
Source | EPA DSSTox | |
URL | https://comptox.epa.gov/dashboard/DTXSID40274460 | |
Description | DSSTox provides a high quality public chemistry resource for supporting improved predictive toxicology. | |
Molecular Weight |
464.6 g/mol | |
Details | Computed by PubChem 2.1 (PubChem release 2021.05.07) | |
Source | PubChem | |
URL | https://pubchem.ncbi.nlm.nih.gov | |
Description | Data deposited in or computed by PubChem | |
CAS No. |
1670-26-4 | |
Record name | 2-Amino-3-hydroxyoctadec-4-en-1-yl 2-(trimethylazaniumyl)ethyl phosphate | |
Source | EPA DSSTox | |
URL | https://comptox.epa.gov/dashboard/DTXSID40274460 | |
Description | DSSTox provides a high quality public chemistry resource for supporting improved predictive toxicology. | |
Retrosynthesis Analysis
AI-Powered Synthesis Planning: Our tool employs the Template_relevance Pistachio, Template_relevance Bkms_metabolic, Template_relevance Pistachio_ringbreaker, Template_relevance Reaxys, Template_relevance Reaxys_biocatalysis model, leveraging a vast database of chemical reactions to predict feasible synthetic routes.
One-Step Synthesis Focus: Specifically designed for one-step synthesis, it provides concise and direct routes for your target compounds, streamlining the synthesis process.
Accurate Predictions: Utilizing the extensive PISTACHIO, BKMS_METABOLIC, PISTACHIO_RINGBREAKER, REAXYS, REAXYS_BIOCATALYSIS database, our tool offers high-accuracy predictions, reflecting the latest in chemical research and data.
Strategy Settings
Precursor scoring | Relevance Heuristic |
---|---|
Min. plausibility | 0.01 |
Model | Template_relevance |
Template Set | Pistachio/Bkms_metabolic/Pistachio_ringbreaker/Reaxys/Reaxys_biocatalysis |
Top-N result to add to graph | 6 |
Feasible Synthetic Routes
Disclaimer and Information on In-Vitro Research Products
Please be aware that all articles and product information presented on BenchChem are intended solely for informational purposes. The products available for purchase on BenchChem are specifically designed for in-vitro studies, which are conducted outside of living organisms. In-vitro studies, derived from the Latin term "in glass," involve experiments performed in controlled laboratory settings using cells or tissues. It is important to note that these products are not categorized as medicines or drugs, and they have not received approval from the FDA for the prevention, treatment, or cure of any medical condition, ailment, or disease. We must emphasize that any form of bodily introduction of these products into humans or animals is strictly prohibited by law. It is essential to adhere to these guidelines to ensure compliance with legal and ethical standards in research and experimentation.