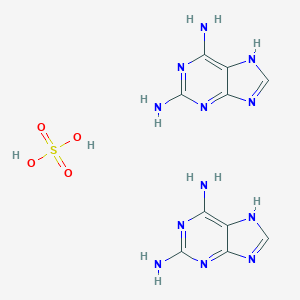
2,6-Diaminopurine hemisulfate salt
Overview
Description
2,6-Diaminopurine hemisulfate salt (2,6-DAP) is an organic salt of the purine base 2,6-diaminopurine. It is a widely used reagent in organic synthesis and has been studied extensively in the field of biochemistry and physiology. 2,6-DAP is a white, crystalline powder with a melting point of 219-220°C. Its solubility in water is 0.05 g/L at 25°C. 2,6-DAP has been shown to be a valuable tool in the synthesis of a variety of complex molecules, and its biochemical and physiological effects have been studied in depth.
Scientific Research Applications
2,6-Diaminopurine forms hydrogen-bonded complexes with certain bases, useful in understanding Watson-Crick and Hoogsteen type base pairing in the solid state (Sakore et al., 1969).
It is a precursor in the synthesis of key nucleosides like 2'-deoxyisoguanosine, essential for adenosine and adenosine-diphosphate complexes (Arico et al., 2010).
It acts as a biocompatible inhibitor of copper corrosion in simulated body fluids, highlighting its potential in biological applications (Mihajlovic et al., 2019).
The crystal structure of its derivatives offers insights into peptidic nucleic acid synthesis (Sood et al., 1997).
2,6-Diaminopurine exhibits inhibitory effects on DNA transcription, suggesting roles in transcriptional regulation (Tan et al., 2022).
Its antiviral properties are evident in its inhibition of vaccinia virus multiplication (Thompson et al., 1950).
It influences root growth in plants, impacting both lateral and main axis meristems (Goldacre & Unt, 1957).
Biocatalysis research uses it for producing nucleosides, vital in prodrug development and antisense oligonucleotide synthesis (De Benedetti et al., 2012).
Mechanism of Action
Target of Action
2,6-Diaminopurine (2,6-DAP), also known as 2-aminoadenine, is an adenine analogue . It is found instead of adenine (A) in the genetic material of some bacteriophage viruses .
Mode of Action
2,6-Diaminopurine can base pair with thymine in DNA, and with uracil in RNA, to give three Watson-Crick hydrogen bonds . This alteration in base pairing properties has led to the use of DAP as a structural probe of molecular recognition between ligands and DNA .
Biochemical Pathways
The S-2L phage avoids incorporating A bases in the genome by hydrolyzing dATP (DatZ enzyme) . The Z base is produced by a pathway involving DUF550 (MazZ) and PurZ in S-2L and Vibrio phage PhiVC8 . The PrimPol/AEP DNA polymerase responsible for handling the Z base occurs in the same gene cluster as the three aforementioned enzymes .
Pharmacokinetics
Its solubility in water is 238 g/L at 20 °C , which may influence its absorption and distribution in the body.
Result of Action
The use of 2,6-Diaminopurine results in an improved stability that affects protein-binding interactions that rely on those differences . This compound was once used in the treatment of leukemia .
Action Environment
The action of 2,6-Diaminopurine can be influenced by environmental factors. For instance, in August 2021, it was shown that DatZ, MazZ, and PurZ are sufficient to replace some occurrence of A by Z in the bacterial genome of E. coli; expression of this system is toxic to the cell .
Safety and Hazards
Future Directions
Properties
IUPAC Name |
7H-purine-2,6-diamine;sulfuric acid | |
---|---|---|
Source | PubChem | |
URL | https://pubchem.ncbi.nlm.nih.gov | |
Description | Data deposited in or computed by PubChem | |
InChI |
InChI=1S/2C5H6N6.H2O4S/c2*6-3-2-4(9-1-8-2)11-5(7)10-3;1-5(2,3)4/h2*1H,(H5,6,7,8,9,10,11);(H2,1,2,3,4) | |
Source | PubChem | |
URL | https://pubchem.ncbi.nlm.nih.gov | |
Description | Data deposited in or computed by PubChem | |
InChI Key |
HNHVJLBUCSUIFH-UHFFFAOYSA-N | |
Source | PubChem | |
URL | https://pubchem.ncbi.nlm.nih.gov | |
Description | Data deposited in or computed by PubChem | |
Canonical SMILES |
C1=NC2=NC(=NC(=C2N1)N)N.C1=NC2=NC(=NC(=C2N1)N)N.OS(=O)(=O)O | |
Source | PubChem | |
URL | https://pubchem.ncbi.nlm.nih.gov | |
Description | Data deposited in or computed by PubChem | |
Molecular Formula |
C10H14N12O4S | |
Source | PubChem | |
URL | https://pubchem.ncbi.nlm.nih.gov | |
Description | Data deposited in or computed by PubChem | |
DSSTOX Substance ID |
DTXSID40989143 | |
Record name | Sulfuric acid--2-imino-2,3-dihydro-1H-purin-6-amine (1/2) | |
Source | EPA DSSTox | |
URL | https://comptox.epa.gov/dashboard/DTXSID40989143 | |
Description | DSSTox provides a high quality public chemistry resource for supporting improved predictive toxicology. | |
Molecular Weight |
398.37 g/mol | |
Source | PubChem | |
URL | https://pubchem.ncbi.nlm.nih.gov | |
Description | Data deposited in or computed by PubChem | |
CAS No. |
69369-16-0 | |
Record name | 1H-Purine-2,6-diamine, sulfate (2:1) | |
Source | ChemIDplus | |
URL | https://pubchem.ncbi.nlm.nih.gov/substance/?source=chemidplus&sourceid=0069369160 | |
Description | ChemIDplus is a free, web search system that provides access to the structure and nomenclature authority files used for the identification of chemical substances cited in National Library of Medicine (NLM) databases, including the TOXNET system. | |
Record name | Sulfuric acid--2-imino-2,3-dihydro-1H-purin-6-amine (1/2) | |
Source | EPA DSSTox | |
URL | https://comptox.epa.gov/dashboard/DTXSID40989143 | |
Description | DSSTox provides a high quality public chemistry resource for supporting improved predictive toxicology. | |
Retrosynthesis Analysis
AI-Powered Synthesis Planning: Our tool employs the Template_relevance Pistachio, Template_relevance Bkms_metabolic, Template_relevance Pistachio_ringbreaker, Template_relevance Reaxys, Template_relevance Reaxys_biocatalysis model, leveraging a vast database of chemical reactions to predict feasible synthetic routes.
One-Step Synthesis Focus: Specifically designed for one-step synthesis, it provides concise and direct routes for your target compounds, streamlining the synthesis process.
Accurate Predictions: Utilizing the extensive PISTACHIO, BKMS_METABOLIC, PISTACHIO_RINGBREAKER, REAXYS, REAXYS_BIOCATALYSIS database, our tool offers high-accuracy predictions, reflecting the latest in chemical research and data.
Strategy Settings
Precursor scoring | Relevance Heuristic |
---|---|
Min. plausibility | 0.01 |
Model | Template_relevance |
Template Set | Pistachio/Bkms_metabolic/Pistachio_ringbreaker/Reaxys/Reaxys_biocatalysis |
Top-N result to add to graph | 6 |
Feasible Synthetic Routes
Disclaimer and Information on In-Vitro Research Products
Please be aware that all articles and product information presented on BenchChem are intended solely for informational purposes. The products available for purchase on BenchChem are specifically designed for in-vitro studies, which are conducted outside of living organisms. In-vitro studies, derived from the Latin term "in glass," involve experiments performed in controlled laboratory settings using cells or tissues. It is important to note that these products are not categorized as medicines or drugs, and they have not received approval from the FDA for the prevention, treatment, or cure of any medical condition, ailment, or disease. We must emphasize that any form of bodily introduction of these products into humans or animals is strictly prohibited by law. It is essential to adhere to these guidelines to ensure compliance with legal and ethical standards in research and experimentation.