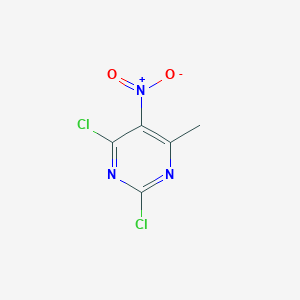
2,4-Dichloro-6-methyl-5-nitropyrimidine
Overview
Description
2,4-Dichloro-6-methyl-5-nitropyrimidine is a synthetic intermediate compound with the molecular formula C5H3Cl2N3O2 and a molecular weight of 208.00 g/mol . It is primarily used in research and industrial applications due to its unique chemical properties and reactivity.
Preparation Methods
Synthetic Routes and Reaction Conditions: The synthesis of 2,4-Dichloro-6-methyl-5-nitropyrimidine typically involves the cyclization of acetamidine hydrochloride and diethyl malonate in the presence of sodium methoxide, yielding 4,6-dihydro-2-methyl-pyrimidine. This intermediate is then nitrated using a mixture of nitric acid, trichloroacetic acid, and acetic acid to produce 4,6-dihydro-2-methyl-5-nitropyrimidine. Finally, chlorination with phosphorus oxytrichloride results in the formation of this compound .
Industrial Production Methods: Industrial production methods for this compound are similar to laboratory synthesis but are scaled up to accommodate larger quantities. The process involves stringent control of reaction conditions to ensure high yield and purity.
Chemical Reactions Analysis
Types of Reactions: 2,4-Dichloro-6-methyl-5-nitropyrimidine undergoes various chemical reactions, including:
Substitution Reactions: It can participate in nucleophilic substitution reactions due to the presence of chlorine atoms.
Reduction Reactions: The nitro group can be reduced to an amino group under appropriate conditions.
Oxidation Reactions: The methyl group can be oxidized to a carboxyl group.
Common Reagents and Conditions:
Substitution: Reagents such as sodium methoxide or potassium tert-butoxide in an aprotic solvent.
Reduction: Catalytic hydrogenation using palladium on carbon or chemical reduction using iron and hydrochloric acid.
Oxidation: Potassium permanganate or chromium trioxide in acidic conditions.
Major Products:
Substitution: Formation of various substituted pyrimidines.
Reduction: Formation of 2,4-Dichloro-6-methyl-5-aminopyrimidine.
Oxidation: Formation of 2,4-Dichloro-6-carboxy-5-nitropyrimidine.
Scientific Research Applications
2,4-Dichloro-6-methyl-5-nitropyrimidine is utilized in various scientific research applications:
Chemistry: As a building block for the synthesis of more complex pyrimidine derivatives.
Biology: Used in the study of enzyme interactions and as a potential antibacterial agent.
Industry: Employed in the production of agrochemicals and pharmaceuticals.
Mechanism of Action
The mechanism of action of 2,4-Dichloro-6-methyl-5-nitropyrimidine involves its interaction with bacterial ribosomal RNA, inhibiting protein synthesis and thereby preventing bacterial growth . It also exhibits antioxidant activity, which contributes to its antibacterial properties.
Comparison with Similar Compounds
- 2,4-Dichloro-5-nitropyrimidine
- 2,4-Dihydroxy-6-methyl-5-nitropyrimidine
- 4,6-Dichloro-2-methyl-5-nitropyrimidine
Comparison: 2,4-Dichloro-6-methyl-5-nitropyrimidine is unique due to its specific substitution pattern, which imparts distinct chemical reactivity and biological activity. Compared to similar compounds, it has a higher degree of chlorination, which enhances its ability to undergo nucleophilic substitution reactions and increases its antibacterial efficacy .
Properties
IUPAC Name |
2,4-dichloro-6-methyl-5-nitropyrimidine | |
---|---|---|
Source | PubChem | |
URL | https://pubchem.ncbi.nlm.nih.gov | |
Description | Data deposited in or computed by PubChem | |
InChI |
InChI=1S/C5H3Cl2N3O2/c1-2-3(10(11)12)4(6)9-5(7)8-2/h1H3 | |
Source | PubChem | |
URL | https://pubchem.ncbi.nlm.nih.gov | |
Description | Data deposited in or computed by PubChem | |
InChI Key |
NBCOZXBHPKSFSA-UHFFFAOYSA-N | |
Source | PubChem | |
URL | https://pubchem.ncbi.nlm.nih.gov | |
Description | Data deposited in or computed by PubChem | |
Canonical SMILES |
CC1=C(C(=NC(=N1)Cl)Cl)[N+](=O)[O-] | |
Source | PubChem | |
URL | https://pubchem.ncbi.nlm.nih.gov | |
Description | Data deposited in or computed by PubChem | |
Molecular Formula |
C5H3Cl2N3O2 | |
Source | PubChem | |
URL | https://pubchem.ncbi.nlm.nih.gov | |
Description | Data deposited in or computed by PubChem | |
DSSTOX Substance ID |
DTXSID10298254 | |
Record name | 2,4-Dichloro-6-methyl-5-nitropyrimidine | |
Source | EPA DSSTox | |
URL | https://comptox.epa.gov/dashboard/DTXSID10298254 | |
Description | DSSTox provides a high quality public chemistry resource for supporting improved predictive toxicology. | |
Molecular Weight |
208.00 g/mol | |
Source | PubChem | |
URL | https://pubchem.ncbi.nlm.nih.gov | |
Description | Data deposited in or computed by PubChem | |
CAS No. |
13162-26-0 | |
Record name | 13162-26-0 | |
Source | DTP/NCI | |
URL | https://dtp.cancer.gov/dtpstandard/servlet/dwindex?searchtype=NSC&outputformat=html&searchlist=122005 | |
Description | The NCI Development Therapeutics Program (DTP) provides services and resources to the academic and private-sector research communities worldwide to facilitate the discovery and development of new cancer therapeutic agents. | |
Explanation | Unless otherwise indicated, all text within NCI products is free of copyright and may be reused without our permission. Credit the National Cancer Institute as the source. | |
Record name | 2,4-Dichloro-6-methyl-5-nitropyrimidine | |
Source | EPA DSSTox | |
URL | https://comptox.epa.gov/dashboard/DTXSID10298254 | |
Description | DSSTox provides a high quality public chemistry resource for supporting improved predictive toxicology. | |
Record name | 2,4-dichloro-6-methyl-5-nitropyrimidine | |
Source | European Chemicals Agency (ECHA) | |
URL | https://echa.europa.eu/information-on-chemicals | |
Description | The European Chemicals Agency (ECHA) is an agency of the European Union which is the driving force among regulatory authorities in implementing the EU's groundbreaking chemicals legislation for the benefit of human health and the environment as well as for innovation and competitiveness. | |
Explanation | Use of the information, documents and data from the ECHA website is subject to the terms and conditions of this Legal Notice, and subject to other binding limitations provided for under applicable law, the information, documents and data made available on the ECHA website may be reproduced, distributed and/or used, totally or in part, for non-commercial purposes provided that ECHA is acknowledged as the source: "Source: European Chemicals Agency, http://echa.europa.eu/". Such acknowledgement must be included in each copy of the material. ECHA permits and encourages organisations and individuals to create links to the ECHA website under the following cumulative conditions: Links can only be made to webpages that provide a link to the Legal Notice page. | |
Retrosynthesis Analysis
AI-Powered Synthesis Planning: Our tool employs the Template_relevance Pistachio, Template_relevance Bkms_metabolic, Template_relevance Pistachio_ringbreaker, Template_relevance Reaxys, Template_relevance Reaxys_biocatalysis model, leveraging a vast database of chemical reactions to predict feasible synthetic routes.
One-Step Synthesis Focus: Specifically designed for one-step synthesis, it provides concise and direct routes for your target compounds, streamlining the synthesis process.
Accurate Predictions: Utilizing the extensive PISTACHIO, BKMS_METABOLIC, PISTACHIO_RINGBREAKER, REAXYS, REAXYS_BIOCATALYSIS database, our tool offers high-accuracy predictions, reflecting the latest in chemical research and data.
Strategy Settings
Precursor scoring | Relevance Heuristic |
---|---|
Min. plausibility | 0.01 |
Model | Template_relevance |
Template Set | Pistachio/Bkms_metabolic/Pistachio_ringbreaker/Reaxys/Reaxys_biocatalysis |
Top-N result to add to graph | 6 |
Feasible Synthetic Routes
Q1: What makes 2,4-Dichloro-6-methyl-5-nitropyrimidine a useful starting material in organic synthesis?
A: This compound possesses three chlorine atoms and a nitro group, all of which are susceptible to nucleophilic substitution reactions. [, , ] This makes it a highly versatile building block for creating a variety of substituted pyrimidine derivatives, which are often found in biologically active compounds and pharmaceuticals.
Q2: Can you describe a specific example of how this compound has been modified to create new compounds with potential biological activity?
A: One research group utilized Suzuki-Miyaura coupling reactions to replace the chlorine atoms in this compound with various aryl groups. [] This resulted in a series of novel 2,4-diaryl-6-methyl-5-nitropyrimidines that exhibited promising antibacterial and antioxidant properties in preliminary screenings.
Q3: Apart from Suzuki-Miyaura coupling, what other types of reactions has this compound been used in?
A: This compound has proven useful in constructing more complex heterocyclic systems. For example, researchers successfully synthesized a series of oxazolo[5,4-d][1,2,4]triazolo[4,3-a]pyrimidines, a novel class of heterocycles, starting from this compound. [] The synthetic route involved a series of reactions including nucleophilic substitution with aryl carboxylic acids, hydrazine cyclization, and finally ring closure using triethylorthoesters or carbondisulfide and alkylhalides.
Q4: Has this compound been studied for its potential to form imidazopyrimidine derivatives?
A: Yes, research has shown that reacting this compound with 2-chloroethylamine can lead to the formation of tetrahydrodi-imidazopyrimidine derivatives. [] This reaction highlights the versatility of the starting compound in accessing a range of valuable nitrogen-containing heterocycles.
Disclaimer and Information on In-Vitro Research Products
Please be aware that all articles and product information presented on BenchChem are intended solely for informational purposes. The products available for purchase on BenchChem are specifically designed for in-vitro studies, which are conducted outside of living organisms. In-vitro studies, derived from the Latin term "in glass," involve experiments performed in controlled laboratory settings using cells or tissues. It is important to note that these products are not categorized as medicines or drugs, and they have not received approval from the FDA for the prevention, treatment, or cure of any medical condition, ailment, or disease. We must emphasize that any form of bodily introduction of these products into humans or animals is strictly prohibited by law. It is essential to adhere to these guidelines to ensure compliance with legal and ethical standards in research and experimentation.