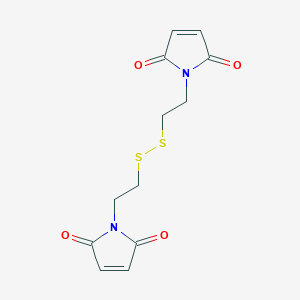
Dithiobis(N-ethylmaleimide)
Overview
Description
Dithiobis(N-ethylmaleimide) (DNB) is an organic compound that has been used as an important tool in biochemical and physiological research for over 40 years. It is a thiol-reactive reagent that has many potential applications in biochemical and physiological research. DNB is a thiol-reactive reagent that binds to the thiol groups of proteins, peptides, and other molecules, thus allowing for the detection, isolation, and characterization of these molecules.
Scientific Research Applications
Protein Labeling & Crosslinking
DTME is used in protein labeling and crosslinking . It forms a covalent bond with sulfhydryl groups, such as those found in cysteine residues of proteins or peptides . This allows for the study of protein-protein interactions and the structure of protein complexes .
Protein Structure Analysis
DTME is commonly used to explore and characterize protein structure . It can help determine the oligomerization state of proteins, which is crucial for understanding protein function .
Reversible Conjugation
The presence of a disulfide bond in the center of the spacer arm of DTME allows for reversible covalent conjugation . This means that the crosslinks formed by DTME can be cleaved with a reducing agent such as dithiothreitol (DTT), allowing for the reversible modification of proteins .
Drug Delivery Research
The reversible nature of the DTME crosslinking can be exploited in drug delivery research. The disulfide bond in DTME is stable in extracellular conditions but can be cleaved in the reducing environment of the cell, potentially releasing a conjugated drug .
Bioconjugation in Material Science
DTME can be used in the field of material science for bioconjugation. It can be used to attach biomolecules to surfaces, creating bioactive materials for various applications, including biosensors and bioactive implants .
Study of Protein Interactions
DTME is used to study protein interactions . By crosslinking proteins, researchers can stabilize transient or weak interactions, allowing them to be studied in more detail .
Mechanism of Action
Target of Action
DTME is a homobifunctional, maleimide crosslinker . Its primary targets are sulfhydryl groups (-SH) , typically found in cysteine residues of proteins or peptides . These groups play a crucial role in protein structure and function, and their modification can significantly impact protein activity.
Mode of Action
DTME interacts with its targets through a process known as crosslinking . It forms covalent bonds with sulfhydryl groups on two separate molecules, linking them together . This crosslinking can alter the structure and function of the target proteins. The crosslinks formed by DTME are reversible , meaning they can be cleaved or broken apart under certain conditions, such as the presence of a reducing agent like dithiothreitol (DTT) .
Biochemical Pathways
DTME is commonly used to explore and characterize protein structure (i.e., oligomerization) or protein interactions . By crosslinking proteins, DTME can induce or stabilize protein-protein interactions, leading to the formation of protein complexes. These complexes can then participate in various biochemical pathways, with the specific effects depending on the proteins involved.
Pharmacokinetics
It’s important to note that dtme iswater-insoluble . It must first be dissolved in an organic solvent like DMF or DMSO before it can be added to aqueous reaction buffers . This property could potentially affect its bioavailability and distribution within a biological system.
Result of Action
The molecular and cellular effects of DTME’s action are largely dependent on the specific proteins it targets. By crosslinking proteins, DTME can induce changes in protein conformation, disrupt protein-protein interactions, or stabilize protein complexes . These changes can have wide-ranging effects on cellular processes, from signal transduction to gene expression.
Action Environment
DTME is sensitive to moisture . It should be stored in a desiccated condition to maintain its reactivity . Additionally, the crosslinks formed by DTME can be cleaved by reducing agents . Therefore, the presence of such agents in the environment can influence the stability and efficacy of DTME’s action. Lastly, as DTME is water-insoluble, the solvent used to dissolve it can also impact its action .
properties
IUPAC Name |
1-[2-[2-(2,5-dioxopyrrol-1-yl)ethyldisulfanyl]ethyl]pyrrole-2,5-dione | |
---|---|---|
Source | PubChem | |
URL | https://pubchem.ncbi.nlm.nih.gov | |
Description | Data deposited in or computed by PubChem | |
InChI |
InChI=1S/C12H12N2O4S2/c15-9-1-2-10(16)13(9)5-7-19-20-8-6-14-11(17)3-4-12(14)18/h1-4H,5-8H2 | |
Source | PubChem | |
URL | https://pubchem.ncbi.nlm.nih.gov | |
Description | Data deposited in or computed by PubChem | |
InChI Key |
SGVWDRVQIYUSRA-UHFFFAOYSA-N | |
Source | PubChem | |
URL | https://pubchem.ncbi.nlm.nih.gov | |
Description | Data deposited in or computed by PubChem | |
Canonical SMILES |
C1=CC(=O)N(C1=O)CCSSCCN2C(=O)C=CC2=O | |
Source | PubChem | |
URL | https://pubchem.ncbi.nlm.nih.gov | |
Description | Data deposited in or computed by PubChem | |
Molecular Formula |
C12H12N2O4S2 | |
Source | PubChem | |
URL | https://pubchem.ncbi.nlm.nih.gov | |
Description | Data deposited in or computed by PubChem | |
DSSTOX Substance ID |
DTXSID70222136 | |
Record name | Dithiobis(N-ethylmaleimide) | |
Source | EPA DSSTox | |
URL | https://comptox.epa.gov/dashboard/DTXSID70222136 | |
Description | DSSTox provides a high quality public chemistry resource for supporting improved predictive toxicology. | |
Molecular Weight |
312.4 g/mol | |
Source | PubChem | |
URL | https://pubchem.ncbi.nlm.nih.gov | |
Description | Data deposited in or computed by PubChem | |
Product Name |
Dithiobis(N-ethylmaleimide) | |
CAS RN |
71865-37-7 | |
Record name | Dithiobis(N-ethylmaleimide) | |
Source | ChemIDplus | |
URL | https://pubchem.ncbi.nlm.nih.gov/substance/?source=chemidplus&sourceid=0071865377 | |
Description | ChemIDplus is a free, web search system that provides access to the structure and nomenclature authority files used for the identification of chemical substances cited in National Library of Medicine (NLM) databases, including the TOXNET system. | |
Record name | Dithiobis(N-ethylmaleimide) | |
Source | EPA DSSTox | |
URL | https://comptox.epa.gov/dashboard/DTXSID70222136 | |
Description | DSSTox provides a high quality public chemistry resource for supporting improved predictive toxicology. | |
Record name | Bis(2-maleimidoethyl) Disulfide | |
Source | European Chemicals Agency (ECHA) | |
URL | https://echa.europa.eu/information-on-chemicals | |
Description | The European Chemicals Agency (ECHA) is an agency of the European Union which is the driving force among regulatory authorities in implementing the EU's groundbreaking chemicals legislation for the benefit of human health and the environment as well as for innovation and competitiveness. | |
Explanation | Use of the information, documents and data from the ECHA website is subject to the terms and conditions of this Legal Notice, and subject to other binding limitations provided for under applicable law, the information, documents and data made available on the ECHA website may be reproduced, distributed and/or used, totally or in part, for non-commercial purposes provided that ECHA is acknowledged as the source: "Source: European Chemicals Agency, http://echa.europa.eu/". Such acknowledgement must be included in each copy of the material. ECHA permits and encourages organisations and individuals to create links to the ECHA website under the following cumulative conditions: Links can only be made to webpages that provide a link to the Legal Notice page. | |
Retrosynthesis Analysis
AI-Powered Synthesis Planning: Our tool employs the Template_relevance Pistachio, Template_relevance Bkms_metabolic, Template_relevance Pistachio_ringbreaker, Template_relevance Reaxys, Template_relevance Reaxys_biocatalysis model, leveraging a vast database of chemical reactions to predict feasible synthetic routes.
One-Step Synthesis Focus: Specifically designed for one-step synthesis, it provides concise and direct routes for your target compounds, streamlining the synthesis process.
Accurate Predictions: Utilizing the extensive PISTACHIO, BKMS_METABOLIC, PISTACHIO_RINGBREAKER, REAXYS, REAXYS_BIOCATALYSIS database, our tool offers high-accuracy predictions, reflecting the latest in chemical research and data.
Strategy Settings
Precursor scoring | Relevance Heuristic |
---|---|
Min. plausibility | 0.01 |
Model | Template_relevance |
Template Set | Pistachio/Bkms_metabolic/Pistachio_ringbreaker/Reaxys/Reaxys_biocatalysis |
Top-N result to add to graph | 6 |
Feasible Synthetic Routes
Disclaimer and Information on In-Vitro Research Products
Please be aware that all articles and product information presented on BenchChem are intended solely for informational purposes. The products available for purchase on BenchChem are specifically designed for in-vitro studies, which are conducted outside of living organisms. In-vitro studies, derived from the Latin term "in glass," involve experiments performed in controlled laboratory settings using cells or tissues. It is important to note that these products are not categorized as medicines or drugs, and they have not received approval from the FDA for the prevention, treatment, or cure of any medical condition, ailment, or disease. We must emphasize that any form of bodily introduction of these products into humans or animals is strictly prohibited by law. It is essential to adhere to these guidelines to ensure compliance with legal and ethical standards in research and experimentation.