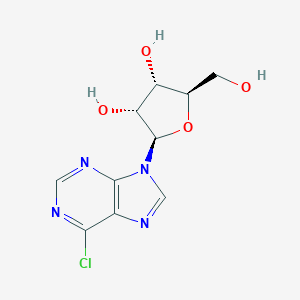
6-Chloropurine riboside
Overview
Description
6-Chloropurine riboside (6-CPR) is a synthetic nucleoside analog characterized by a chlorine atom at the C6 position of the purine base and a ribose sugar moiety. It serves as a versatile intermediate in medicinal chemistry, enabling the synthesis of bioactive nucleoside derivatives through modifications at the C6 position .
Preparation Methods
Deacetylation of 2,3,5-Tri-O-Acetyl-6-Chloropurine Ribofuranoside
Reaction Mechanism and Conditions
The most widely reported synthesis of 6-CPR involves deacetylation of 2,3,5-tri-O-acetyl-6-chloropurine-9-β-D-ribofuranoside (CAS 2004-06-0) using sodium methoxide in anhydrous methanol . The reaction proceeds via nucleophilic cleavage of acetyl groups at the 2', 3', and 5' positions of the ribose moiety.
In a representative procedure, 532 g of the acetylated precursor is dissolved in 2.43 L of dry methanol under inert conditions . The solution is cooled to −11°C, and 13.2 g of sodium methoxide is added incrementally to prevent exothermic side reactions. After 1 hour of stirring at low temperature, the mixture is warmed to 20–25°C for 2 hours to ensure complete deprotection. The crude product is filtered and washed with cold methanol, yielding 304.6 g (86.4%) of 6-CPR as a light-yellow solid .
Optimization and Scalability
Key factors influencing yield include:
-
Temperature Control : Maintaining subzero temperatures during methoxide addition minimizes purine ring degradation.
-
Solvent Purity : Anhydrous methanol (<0.01% H₂O) prevents hydrolysis of the chloropurine moiety.
-
Stoichiometry : A 1:1.05 molar ratio of precursor to NaOMe ensures complete deacetylation without overbase conditions.
Industrial-scale adaptations replace batch cooling with continuous flow reactors, reducing reaction time to 45 minutes while maintaining >80% yield .
One-Pot Glycosylation-Cyclization from Chloropyrimidines
Methodology Overview
A convergent synthesis route employs amidinoaminochloropyrimidines and 1-O-acetyl-2,3,5-tri-O-benzoyl-β-D-ribose under trimethylsilyl trifluoromethanesulfonate (TMSOTf) catalysis . This method enables simultaneous glycosylation and cyclization, bypassing intermediate isolation.
Stepwise Analysis
-
Precursor Preparation : 4,6-Dichloro-5-nitropyrimidine is treated with ammonium acetate in ethanol at 60°C to yield 4-amino-6-chloro-5-nitropyrimidine.
-
Glycosylation : TMSOTf (1.2 equiv) activates the ribose donor, facilitating coupling with the pyrimidine at −40°C in dichloromethane.
-
Cyclization : Heating to 80°C in acetonitrile induces purine ring formation via intramolecular nucleophilic attack.
The one-pot process achieves 68–72% yield across 10 substrates, with electron-withdrawing groups on pyrimidines enhancing cyclization efficiency .
Enzymatic Deprotection of Chlorinated Guanosine Derivatives
Synthesis from Peracetylated Guanosine
A biocatalytic approach starts with peracetylated guanosine (2',3',5'-tri-O-acetylguanosine), which undergoes chlorination using phosphorus oxychloride (POCl₃) in acetonitrile . The reaction replaces the 6-oxo group with chlorine, yielding 2-amino-6-chloro-2',3',5'-tri-O-acetylpurine riboside quantitatively .
Deprotection Challenges
Initial attempts to remove acetyl groups via acid (HCl/MeOH) or base (K₂CO₃/MeOH) hydrolysis failed due to:
-
Glycosidic Bond Cleavage : Acidic conditions (pH < 3) hydrolyze the β-N-glycosidic linkage .
-
Methoxy Byproduct Formation : Basic conditions induce nucleophilic substitution at C6, generating 6-methoxy derivatives .
Lipase-Mediated Deprotection
Novozyme 435 (Candida antarctica lipase B) in phosphate buffer (pH 8.0, 30 mM) selectively hydrolyzes acetyl groups at 60°C over 8 days . This method preserves the chloropurine structure, affording 6-CPR in 55% yield with >98% purity by HPLC .
Comparative Analysis of Synthetic Routes
Challenges and Innovations
Stability of 6-CPR
The compound degrades above 163°C, necessitating storage at −20°C under argon . Moisture induces hydrolysis to 6-hydroxypurine riboside, mitigated by lyophilization .
Green Chemistry Advances
Recent protocols replace toxic solvents (MeCN, CH₂Cl₂) with cyclopentyl methyl ether (CPME), reducing environmental impact without sacrificing yield .
Chemical Reactions Analysis
Types of Reactions: 6-Chloropurine riboside undergoes various chemical reactions, including:
Substitution Reactions: The chlorine atom at the 6th position can be substituted with other nucleophiles, such as amines or thiols, under appropriate conditions.
Oxidation and Reduction Reactions: The ribose moiety can undergo oxidation to form ribonolactone derivatives or reduction to form deoxyribose derivatives.
Common Reagents and Conditions:
Substitution Reactions: Common reagents include amines, thiols, and other nucleophiles.
Oxidation Reactions: Reagents like potassium permanganate or chromium trioxide can be used under acidic or basic conditions.
Major Products:
Substitution Reactions: Products include various 6-substituted purine ribosides, depending on the nucleophile used.
Oxidation Reactions: Products include ribonolactone derivatives.
Scientific Research Applications
Biochemical Research
Substrate Specificity Studies
6-Chloropurine riboside is primarily utilized to study the kinetics and substrate specificity of enzymes such as adenosine deaminase. The compound serves as a substrate to investigate enzyme activity, allowing researchers to understand the mechanisms underlying nucleoside metabolism. For instance, benzoylation of 6-CPR has been employed to enhance its reactivity in these studies, facilitating detailed kinetic analyses .
Antiviral Research
Potential Antiviral Agents
Research indicates that 6-CPR exhibits antiviral properties, particularly against viruses such as Hepatitis C and coronaviruses. In a study focused on synthesizing nucleoside analogs for antiviral applications, 6-CPR was identified as a lead compound due to its structural similarities to natural nucleosides, which can interfere with viral replication processes .
Agricultural Applications
Plant Pathogen Management
Recent studies have explored the efficacy of this compound in managing plant diseases. Specifically, it has been tested against Candidatus Liberibacter asiaticus (CLas), the bacterium responsible for Huanglongbing (HLB) in citrus plants. Experimental results showed that 6-CPR reduced CLas content significantly in infected leaves, suggesting its potential as a therapeutic agent in agriculture .
Synthesis of Nucleoside Derivatives
Chemical Synthesis
This compound serves as a precursor in the synthesis of various adenosine derivatives with antiproliferative activity. These derivatives are crucial for developing new therapeutic agents targeting cancer and other proliferative diseases. The compound's halogenated structure allows for modifications that enhance biological activity while retaining nucleoside characteristics .
Case Studies and Research Findings
Mechanism of Action
The mechanism of action of 6-chloropurine riboside involves its incorporation into nucleic acids, where it can interfere with DNA and RNA synthesis. It acts as a substrate analog for enzymes involved in nucleoside metabolism, such as adenosine deaminase and inosine monophosphate dehydrogenase . By inhibiting these enzymes, it disrupts the synthesis of nucleotides, leading to the inhibition of cell proliferation and induction of apoptosis .
Comparison with Similar Compounds
Comparison with Structural Analogs
Table 1: Structural Analogs of 6-Chloropurine Riboside
Key Findings :
- Adenosine vs. 6-CPR: Despite high structural similarity, 6-CPR’s C6 chlorine enhances electrophilicity, enabling nucleophilic substitutions for diverse N6-alkyl/aryl derivatives .
- 6-Mercaptopurine Riboside : The -SH group confers redox instability, limiting its utility compared to 6-CPR’s stable halogen .
Functional Analogs: IMPDH Inhibitors
Table 2: IMPDH Inhibitors in Antimicrobial Context
Key Findings :
- 6-CPR outperforms mycophenolic acid in modified culture conditions (BSK-II/60% serum and BSK-II/AHN), suggesting superior uptake or phosphorylation by B. burgdorferi .
- 6-CPR’s activity highlights its role as a salvage pathway antimetabolite, disrupting purine recycling in pathogens .
N6-Alkylated/Arylated Derivatives :
- Synthesis: Palladium-catalyzed cross-coupling of 6-CPR with organozinc halides yields 6-alkyl/aryl purine ribosides (70–97% yields) .
- Applications: N6-(p-Sulfophenyl)alkyl derivatives: Water-soluble adenosine agonists with peripheral selectivity . Antiviral fluorinated analogs: N6-benzyladenosines with trifluoromethyl groups show enhanced selectivity against enteroviruses .
Cyclonucleosides :
- Triflation of 6-CPR derivatives (e.g., isopropylidene-protected 6-CPR) yields 8(R),5'-O-cycloribonucleosides, useful in prodrug design .
Challenges :
- Protective group lability : Acetyl groups in 6-CPR precursors undergo partial cleavage during benzylamine substitutions, necessitating stable iso-butyroyl protections .
Contradictions and Limitations
- Inconsistent anticancer activity : While 6-CPR derivatives show promise in leukemia, 6-CPR itself was ineffective against sarcoma 180 growth .
- Enzymatic processing : ATP deaminase preferentially dechlorinates 6-chloropurine ribotide over 6-CPR, suggesting nucleotide phosphorylation is critical for activity .
Biological Activity
6-Chloropurine riboside (6-CPR) is a purine nucleoside analog that has garnered attention for its potential biological activities, particularly in the fields of virology and cancer research. This article provides a comprehensive overview of the biological activity of 6-CPR, including its mechanisms of action, therapeutic applications, and relevant research findings.
Chemical Structure and Properties
This compound has the molecular formula and a molecular weight of approximately 286.67 g/mol. Its structure consists of a ribofuranose sugar linked to a chlorinated purine base, which is crucial for its biological activity. The compound is known to exist in various forms and can be synthesized through different chemical methods .
The biological activity of 6-CPR is primarily attributed to its role as a substrate for various enzymes involved in nucleotide metabolism. It acts as an inhibitor of purine nucleoside phosphorylase (PNP), an enzyme critical for purine salvage pathways. By inhibiting PNP, 6-CPR can disrupt nucleotide synthesis, which may lead to cytotoxic effects in rapidly dividing cells, such as cancer cells or virus-infected cells .
Antiviral Activity
Research has indicated that 6-CPR exhibits antiviral properties, particularly against hepatitis C virus (HCV). A study demonstrated that the compound could be biosynthesized using thermophilic microorganisms, achieving significant conversion rates. This suggests its potential use in developing antiviral therapies . Additionally, the compound has been explored as a precursor for synthesizing more potent antiviral agents through prodrug technology .
Anticancer Potential
The cytotoxic effects of 6-CPR on cancer cells have been documented in several studies. The compound's ability to inhibit cell proliferation is linked to its interference with nucleic acid synthesis. For instance, it has shown promise in inducing apoptosis in various cancer cell lines, making it a candidate for further investigation as an anticancer agent .
Research Findings and Case Studies
- Enzymatic Studies : In enzymatic assays, 6-CPR was found to be a substrate for PNP from E. coli, with varying levels of activity depending on the enzyme source. This highlights its potential utility in biochemical applications and drug design .
- Biosynthesis : A notable study reported the successful biosynthesis of 6-CPR using Geobacillus stearothermophilus, achieving conversion rates of up to 68%. This method offers a sustainable approach to producing nucleoside analogs for therapeutic use .
- Prodrug Development : The synthesis of kinetin riboside from 6-CPR was explored as part of prodrug technology aimed at enhancing cellular uptake and stability. This research indicates the versatility of 6-CPR as a starting material for developing novel therapeutic agents .
Data Summary
Property | Value |
---|---|
Molecular Formula | |
Molecular Weight | 286.67 g/mol |
CAS Number | 5399-87-1 |
Antiviral Activity | Effective against HCV |
Anticancer Activity | Induces apoptosis |
Q & A
Basic Research Questions
Q. What are the key synthetic routes for preparing 6-chloropurine riboside and its derivatives?
- This compound is commonly synthesized via nucleophilic aromatic substitution (NAS) of 6-chloropurine with ribose derivatives. A reproducible 6-step route involves hydrogenolysis of 2-amino-6-chloropurine riboside, followed by N2-protection with a (di-n-butylamino)methyl group for RNA solid-phase synthesis . For N6-alkylated/arylated derivatives, commercially available 2',3',5'-tri-O-acetyl-6-chloropurine riboside undergoes substitution with amines (e.g., benzylamines, aniline) under mild conditions, followed by deprotection .
Q. How can researchers modify the N6 position of this compound for structural diversification?
- The chlorine atom at the C6 position is replaced via NAS with primary/secondary amines (e.g., methylamine, dimethylamine) in ethanol or DMF. For example:
- Reaction with NaSMe in DMF yields 6-thiopurine riboside (96% yield) .
- Thiourea in ethanol produces 6-thioguanosine derivatives (11% yield) .
- Microwave-assisted NAS enhances efficiency, achieving yields of 70–97% for C6-aminated derivatives .
Q. What methodologies are used to evaluate the biological activity of this compound?
- Antimicrobial Activity : Screen against pathogens like Borrelia burgdorferi in modified culture media (e.g., BSK-II/60% or BSK-II/AHN) at concentrations up to 250 μM. Growth inhibition is quantified via replication curves and % reduction in exponential growth .
- Enzyme Inhibition : Assess IMPDH (inosine-5’-monophosphate dehydrogenase) inhibition using kinetic assays (e.g., kinact and Ki determination). Compare with reference inhibitors like 6-chloropurine ribonucleoside monophosphate (Ki = 62.0 μM) .
Advanced Research Questions
Q. How can researchers resolve contradictions in biological activity data for this compound?
- Contradictory results (e.g., efficacy in BSK-II/AHN vs. inactivity in standard BSK-II for B. burgdorferi) arise from culture condition variability. To address this:
- Test compounds in multiple media formulations.
- Perform dose-response analyses (e.g., 125–250 μM) with triplicate replicates.
- Use statistical modeling (e.g., exponential growth curves) to quantify dose-dependent effects .
Q. What strategies are effective for synthesizing this compound-5'-triphosphate and studying its enzymatic interactions?
- Synthesis : Phosphorylate this compound using dimethyl chlorophosphate in pyridine, followed by sequential phosphorylation with POCl3 to yield the triphosphate .
- Applications :
- Inhibit mRNA capping enzyme Cet1 (IC50 = 2 μM for S. cerevisiae GTPase) .
- Study ATP-binding enzymes (e.g., T4 RNA ligase) via competitive binding assays with this compound-5'-triphosphate .
Q. How does this compound serve as a precursor for non-cleavable substrate analogs in enzyme mechanistic studies?
- Example : Adenosine phosphonobutyric acid-2',3',5'-diphosphate (APBADP), a non-hydrolyzable analog, is synthesized by phosphorylating this compound and coupling with 2-amino-4-phosphonobutyric acid. APBADP is used to probe substrate recognition in argininosuccinate lyase (ASL) via UV-Vis spectroscopy and NMR .
Q. What advanced techniques are employed to study structure-activity relationships (SAR) of this compound derivatives?
- Kinetic Analysis : Compare kobs values for IMPDH inhibitors (e.g., 2-FVIMP vs. 6-chloropurine ribonucleoside monophosphate) to determine potency .
- Chemical Proteomics : Modify RNase A with this compound to map enzyme-substrate interactions via residue-specific labeling .
- Computational Modeling : Predict binding affinities of C6-modified derivatives using molecular docking (e.g., against IMPDH active sites) .
Q. Methodological Considerations
- Reproducibility : Use hydrogenolysis (vs. thioguanosine reduction) for consistent N2-protection .
- Deprotection : Optimize mild conditions (e.g., ammonolysis) to preserve acetyl groups during N6-alkylation .
- Data Validation : Cross-reference activity data with orthogonal assays (e.g., HPLC for purity, NMR for structural confirmation) .
Properties
IUPAC Name |
2-(6-chloropurin-9-yl)-5-(hydroxymethyl)oxolane-3,4-diol | |
---|---|---|
Details | Computed by Lexichem TK 2.7.0 (PubChem release 2021.05.07) | |
Source | PubChem | |
URL | https://pubchem.ncbi.nlm.nih.gov | |
Description | Data deposited in or computed by PubChem | |
InChI |
InChI=1S/C10H11ClN4O4/c11-8-5-9(13-2-12-8)15(3-14-5)10-7(18)6(17)4(1-16)19-10/h2-4,6-7,10,16-18H,1H2 | |
Details | Computed by InChI 1.0.6 (PubChem release 2021.05.07) | |
Source | PubChem | |
URL | https://pubchem.ncbi.nlm.nih.gov | |
Description | Data deposited in or computed by PubChem | |
InChI Key |
XHRJGHCQQPETRH-UHFFFAOYSA-N | |
Details | Computed by InChI 1.0.6 (PubChem release 2021.05.07) | |
Source | PubChem | |
URL | https://pubchem.ncbi.nlm.nih.gov | |
Description | Data deposited in or computed by PubChem | |
Canonical SMILES |
C1=NC2=C(C(=N1)Cl)N=CN2C3C(C(C(O3)CO)O)O | |
Details | Computed by OEChem 2.3.0 (PubChem release 2021.05.07) | |
Source | PubChem | |
URL | https://pubchem.ncbi.nlm.nih.gov | |
Description | Data deposited in or computed by PubChem | |
Molecular Formula |
C10H11ClN4O4 | |
Details | Computed by PubChem 2.1 (PubChem release 2021.05.07) | |
Source | PubChem | |
URL | https://pubchem.ncbi.nlm.nih.gov | |
Description | Data deposited in or computed by PubChem | |
DSSTOX Substance ID |
DTXSID30862810 | |
Record name | 6-Chloro-9-pentofuranosyl-9H-purine | |
Source | EPA DSSTox | |
URL | https://comptox.epa.gov/dashboard/DTXSID30862810 | |
Description | DSSTox provides a high quality public chemistry resource for supporting improved predictive toxicology. | |
Molecular Weight |
286.67 g/mol | |
Details | Computed by PubChem 2.1 (PubChem release 2021.05.07) | |
Source | PubChem | |
URL | https://pubchem.ncbi.nlm.nih.gov | |
Description | Data deposited in or computed by PubChem | |
CAS No. |
7596-60-3, 5399-87-1, 2004-06-0 | |
Record name | NSC407185 | |
Source | DTP/NCI | |
URL | https://dtp.cancer.gov/dtpstandard/servlet/dwindex?searchtype=NSC&outputformat=html&searchlist=407185 | |
Description | The NCI Development Therapeutics Program (DTP) provides services and resources to the academic and private-sector research communities worldwide to facilitate the discovery and development of new cancer therapeutic agents. | |
Explanation | Unless otherwise indicated, all text within NCI products is free of copyright and may be reused without our permission. Credit the National Cancer Institute as the source. | |
Record name | 6-Chloropurine riboside | |
Source | DTP/NCI | |
URL | https://dtp.cancer.gov/dtpstandard/servlet/dwindex?searchtype=NSC&outputformat=html&searchlist=4910 | |
Description | The NCI Development Therapeutics Program (DTP) provides services and resources to the academic and private-sector research communities worldwide to facilitate the discovery and development of new cancer therapeutic agents. | |
Explanation | Unless otherwise indicated, all text within NCI products is free of copyright and may be reused without our permission. Credit the National Cancer Institute as the source. | |
Record name | 6-chloro-9-ribofuranosyl-9H-purine | |
Source | European Chemicals Agency (ECHA) | |
URL | https://echa.europa.eu/substance-information/-/substanceinfo/100.016.278 | |
Description | The European Chemicals Agency (ECHA) is an agency of the European Union which is the driving force among regulatory authorities in implementing the EU's groundbreaking chemicals legislation for the benefit of human health and the environment as well as for innovation and competitiveness. | |
Explanation | Use of the information, documents and data from the ECHA website is subject to the terms and conditions of this Legal Notice, and subject to other binding limitations provided for under applicable law, the information, documents and data made available on the ECHA website may be reproduced, distributed and/or used, totally or in part, for non-commercial purposes provided that ECHA is acknowledged as the source: "Source: European Chemicals Agency, http://echa.europa.eu/". Such acknowledgement must be included in each copy of the material. ECHA permits and encourages organisations and individuals to create links to the ECHA website under the following cumulative conditions: Links can only be made to webpages that provide a link to the Legal Notice page. | |
Retrosynthesis Analysis
AI-Powered Synthesis Planning: Our tool employs the Template_relevance Pistachio, Template_relevance Bkms_metabolic, Template_relevance Pistachio_ringbreaker, Template_relevance Reaxys, Template_relevance Reaxys_biocatalysis model, leveraging a vast database of chemical reactions to predict feasible synthetic routes.
One-Step Synthesis Focus: Specifically designed for one-step synthesis, it provides concise and direct routes for your target compounds, streamlining the synthesis process.
Accurate Predictions: Utilizing the extensive PISTACHIO, BKMS_METABOLIC, PISTACHIO_RINGBREAKER, REAXYS, REAXYS_BIOCATALYSIS database, our tool offers high-accuracy predictions, reflecting the latest in chemical research and data.
Strategy Settings
Precursor scoring | Relevance Heuristic |
---|---|
Min. plausibility | 0.01 |
Model | Template_relevance |
Template Set | Pistachio/Bkms_metabolic/Pistachio_ringbreaker/Reaxys/Reaxys_biocatalysis |
Top-N result to add to graph | 6 |
Feasible Synthetic Routes
Disclaimer and Information on In-Vitro Research Products
Please be aware that all articles and product information presented on BenchChem are intended solely for informational purposes. The products available for purchase on BenchChem are specifically designed for in-vitro studies, which are conducted outside of living organisms. In-vitro studies, derived from the Latin term "in glass," involve experiments performed in controlled laboratory settings using cells or tissues. It is important to note that these products are not categorized as medicines or drugs, and they have not received approval from the FDA for the prevention, treatment, or cure of any medical condition, ailment, or disease. We must emphasize that any form of bodily introduction of these products into humans or animals is strictly prohibited by law. It is essential to adhere to these guidelines to ensure compliance with legal and ethical standards in research and experimentation.