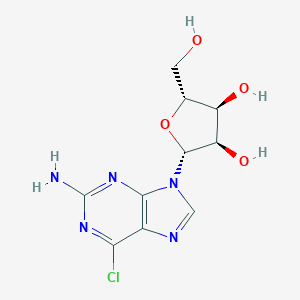
6-Chloroguanosine
Overview
Description
6-Chloroguanineriboside: is a purine nucleoside analogue, specifically a halo nucleoside. It is characterized by the presence of a chlorine atom at the 6th position of the guanine base, which is attached to a ribose sugar. The molecular formula of 6-Chloroguanineriboside is C10H12ClN5O4, and it has a molecular weight of 301.69 g/mol . This compound is primarily used in scientific research due to its unique chemical properties and potential biological activities.
Scientific Research Applications
6-Chloroguanineriboside has a wide range of applications in scientific research, including:
Chemistry: It is used as a building block in the synthesis of more complex molecules and as a reagent in various chemical reactions.
Biology: The compound is studied for its potential biological activities, including its effects on cellular processes and its interactions with biomolecules.
Medicine: Research is ongoing to explore its potential therapeutic applications, particularly in the treatment of certain cancers and viral infections.
Industry: It is used in the development of new materials and as a reference compound in analytical chemistry
Mechanism of Action
Target of Action
6-Chloroguanosine, also known as 2-Amino-6-chloropurine-9-riboside, is a purine nucleoside analogue . Its primary targets are indolent lymphoid malignancies . These are a group of slow-growing lymphomas and leukemias, which include diseases such as chronic lymphocytic leukemia and follicular lymphoma .
Mode of Action
The compound interacts with its targets by inhibiting DNA synthesis and inducing apoptosis . The inhibition of DNA synthesis prevents the replication of cancer cells, thereby slowing down the progression of the disease . The induction of apoptosis, or programmed cell death, leads to the elimination of cancer cells .
Biochemical Pathways
It is known that the compound’s antitumor activity relies on the inhibition of dna synthesis and the induction of apoptosis . These processes are part of the cell cycle and DNA damage pathways .
Pharmacokinetics
It is known that most drug metabolism is achieved by glucuronidation . This process involves the addition of a glucuronic acid group to the drug, making it more water-soluble and easier to excrete from the body .
Result of Action
The result of this compound’s action is the reduction in the number of cancer cells due to the inhibition of DNA synthesis and the induction of apoptosis . This leads to a slowdown in the progression of indolent lymphoid malignancies .
Action Environment
The action of this compound can be influenced by various environmental factors. For instance, the pH of the environment can affect the stability of the compound . .
Safety and Hazards
Biochemical Analysis
Biochemical Properties
6-Chloroguanosine interacts with various enzymes, proteins, and other biomolecules in biochemical reactions . The nature of these interactions involves the inhibition of DNA synthesis and induction of apoptosis . The exact enzymes and proteins it interacts with are not specified in the available literature.
Cellular Effects
This compound has significant effects on various types of cells and cellular processes . It influences cell function by inhibiting DNA synthesis and inducing apoptosis . The impact on cell signaling pathways, gene expression, and cellular metabolism is not specified in the available literature.
Molecular Mechanism
The molecular mechanism of action of this compound involves its binding interactions with biomolecules, enzyme inhibition or activation, and changes in gene expression . It exerts its effects at the molecular level primarily through the inhibition of DNA synthesis and induction of apoptosis .
Dosage Effects in Animal Models
The effects of this compound vary with different dosages in animal models . Specific details about threshold effects observed in these studies, as well as any toxic or adverse effects at high doses, are not specified in the available literature.
Preparation Methods
Synthetic Routes and Reaction Conditions: The synthesis of 6-Chloroguanineriboside typically involves the chlorination of guanosineThe final step involves the deprotection of the hydroxyl groups to yield 6-Chloroguanineriboside .
Industrial Production Methods: Industrial production of 6-Chloroguanineriboside follows similar synthetic routes but on a larger scale. The process involves the use of automated reactors and stringent quality control measures to ensure the purity and consistency of the final product .
Chemical Reactions Analysis
Types of Reactions: 6-Chloroguanineriboside undergoes various chemical reactions, including:
Substitution Reactions: The chlorine atom at the 6th position can be substituted with other nucleophiles.
Oxidation and Reduction Reactions: The compound can undergo oxidation and reduction reactions, altering its chemical structure and properties.
Common Reagents and Conditions:
Substitution Reactions: Common reagents include nucleophiles such as amines and thiols. The reactions are typically carried out in polar solvents under mild conditions.
Oxidation and Reduction Reactions: Reagents such as hydrogen peroxide and sodium borohydride are commonly used.
Major Products: The major products formed from these reactions depend on the specific reagents and conditions used. For example, substitution reactions can yield various derivatives of 6-Chloroguanineriboside with different functional groups .
Comparison with Similar Compounds
6-Chloroguanosine: Similar to 6-Chloroguanineriboside but lacks the ribose sugar.
6-Chloropurine: A simpler analogue with only the purine base and chlorine atom.
6-Thioguanine: Contains a sulfur atom instead of chlorine at the 6th position.
Uniqueness: 6-Chloroguanineriboside is unique due to its specific structure, which includes both the chlorine-substituted guanine base and the ribose sugar. This combination imparts distinct chemical and biological properties, making it valuable for various research applications .
Properties
IUPAC Name |
2-(2-amino-6-chloropurin-9-yl)-5-(hydroxymethyl)oxolane-3,4-diol | |
---|---|---|
Details | Computed by Lexichem TK 2.7.0 (PubChem release 2021.05.07) | |
Source | PubChem | |
URL | https://pubchem.ncbi.nlm.nih.gov | |
Description | Data deposited in or computed by PubChem | |
InChI |
InChI=1S/C10H12ClN5O4/c11-7-4-8(15-10(12)14-7)16(2-13-4)9-6(19)5(18)3(1-17)20-9/h2-3,5-6,9,17-19H,1H2,(H2,12,14,15) | |
Details | Computed by InChI 1.0.6 (PubChem release 2021.05.07) | |
Source | PubChem | |
URL | https://pubchem.ncbi.nlm.nih.gov | |
Description | Data deposited in or computed by PubChem | |
InChI Key |
TXWHPSZYRUHEGT-UHFFFAOYSA-N | |
Details | Computed by InChI 1.0.6 (PubChem release 2021.05.07) | |
Source | PubChem | |
URL | https://pubchem.ncbi.nlm.nih.gov | |
Description | Data deposited in or computed by PubChem | |
Canonical SMILES |
C1=NC2=C(N1C3C(C(C(O3)CO)O)O)N=C(N=C2Cl)N | |
Details | Computed by OEChem 2.3.0 (PubChem release 2021.05.07) | |
Source | PubChem | |
URL | https://pubchem.ncbi.nlm.nih.gov | |
Description | Data deposited in or computed by PubChem | |
Molecular Formula |
C10H12ClN5O4 | |
Details | Computed by PubChem 2.1 (PubChem release 2021.05.07) | |
Source | PubChem | |
URL | https://pubchem.ncbi.nlm.nih.gov | |
Description | Data deposited in or computed by PubChem | |
DSSTOX Substance ID |
DTXSID90942041 | |
Record name | 6-Chloro-9-pentofuranosyl-1,9-dihydro-2H-purin-2-imine | |
Source | EPA DSSTox | |
URL | https://comptox.epa.gov/dashboard/DTXSID90942041 | |
Description | DSSTox provides a high quality public chemistry resource for supporting improved predictive toxicology. | |
Molecular Weight |
301.69 g/mol | |
Details | Computed by PubChem 2.1 (PubChem release 2021.05.07) | |
Source | PubChem | |
URL | https://pubchem.ncbi.nlm.nih.gov | |
Description | Data deposited in or computed by PubChem | |
CAS No. |
34793-10-7, 2004-07-1 | |
Record name | NSC125627 | |
Source | DTP/NCI | |
URL | https://dtp.cancer.gov/dtpstandard/servlet/dwindex?searchtype=NSC&outputformat=html&searchlist=125627 | |
Description | The NCI Development Therapeutics Program (DTP) provides services and resources to the academic and private-sector research communities worldwide to facilitate the discovery and development of new cancer therapeutic agents. | |
Explanation | Unless otherwise indicated, all text within NCI products is free of copyright and may be reused without our permission. Credit the National Cancer Institute as the source. | |
Record name | MLS002608637 | |
Source | DTP/NCI | |
URL | https://dtp.cancer.gov/dtpstandard/servlet/dwindex?searchtype=NSC&outputformat=html&searchlist=44586 | |
Description | The NCI Development Therapeutics Program (DTP) provides services and resources to the academic and private-sector research communities worldwide to facilitate the discovery and development of new cancer therapeutic agents. | |
Explanation | Unless otherwise indicated, all text within NCI products is free of copyright and may be reused without our permission. Credit the National Cancer Institute as the source. | |
Record name | 6-Chloro-9-pentofuranosyl-1,9-dihydro-2H-purin-2-imine | |
Source | EPA DSSTox | |
URL | https://comptox.epa.gov/dashboard/DTXSID90942041 | |
Description | DSSTox provides a high quality public chemistry resource for supporting improved predictive toxicology. | |
Retrosynthesis Analysis
AI-Powered Synthesis Planning: Our tool employs the Template_relevance Pistachio, Template_relevance Bkms_metabolic, Template_relevance Pistachio_ringbreaker, Template_relevance Reaxys, Template_relevance Reaxys_biocatalysis model, leveraging a vast database of chemical reactions to predict feasible synthetic routes.
One-Step Synthesis Focus: Specifically designed for one-step synthesis, it provides concise and direct routes for your target compounds, streamlining the synthesis process.
Accurate Predictions: Utilizing the extensive PISTACHIO, BKMS_METABOLIC, PISTACHIO_RINGBREAKER, REAXYS, REAXYS_BIOCATALYSIS database, our tool offers high-accuracy predictions, reflecting the latest in chemical research and data.
Strategy Settings
Precursor scoring | Relevance Heuristic |
---|---|
Min. plausibility | 0.01 |
Model | Template_relevance |
Template Set | Pistachio/Bkms_metabolic/Pistachio_ringbreaker/Reaxys/Reaxys_biocatalysis |
Top-N result to add to graph | 6 |
Feasible Synthetic Routes
Disclaimer and Information on In-Vitro Research Products
Please be aware that all articles and product information presented on BenchChem are intended solely for informational purposes. The products available for purchase on BenchChem are specifically designed for in-vitro studies, which are conducted outside of living organisms. In-vitro studies, derived from the Latin term "in glass," involve experiments performed in controlled laboratory settings using cells or tissues. It is important to note that these products are not categorized as medicines or drugs, and they have not received approval from the FDA for the prevention, treatment, or cure of any medical condition, ailment, or disease. We must emphasize that any form of bodily introduction of these products into humans or animals is strictly prohibited by law. It is essential to adhere to these guidelines to ensure compliance with legal and ethical standards in research and experimentation.