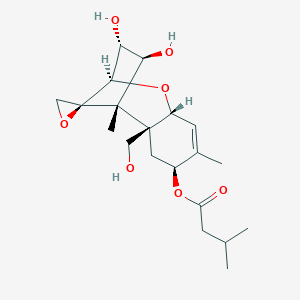
T-2 triol
Overview
Description
- T-2 Triol is a trichothecene mycotoxin derived from the metabolism of T-2 toxin.
- It is less toxic than T-2 toxin .
- Chemical formula:
C20H30O7
. - CAS number: 34114-98-2.
Mechanism of Action
Target of Action
T-2 Triol, also known as T-2 Toxin Triol, is a trichothecene mycotoxin derived from the metabolism of T-2 toxin . It primarily targets the antioxidant defense system of cells, such as HepG2 cells . The primary targets include glutathione (GSH) and the enzymatic activities related to GSH, such as glutathione peroxidase (GPx) and glutathione S-transferase (GST) .
Mode of Action
This compound interacts with its targets, leading to changes in their activities. For instance, exposure to this compound results in an overall decrease in GSH levels . The GSH levels and the enzymatic activities related to GSH (GPx and GST) increase with N-acetylcysteine (NAC) pre-treatment (a glutathione precursor) and decrease with buthionine sulfoximine (BSO) pre-treatment (a glutathione inhibitor) .
Biochemical Pathways
The T-2 toxin and its metabolites, including this compound, affect several biochemical pathways. They can induce oxidative stress, leading to changes in the antioxidant defense system of cells . The GPx activity is increased by T2-tetraol, another metabolite of T-2 toxin . The GST activity increases after T-2 and T2-triol exposure; however, T2-tetraol decreases its activity .
Pharmacokinetics
This compound is rapidly metabolized in the body. In broiler chickens, the half-life (t1/2λz), peak plasma concentrations (Cmax), and Tmax values of this compound are 9.6 mins, 563 ng/ml, and 2.5 mins, respectively . This indicates that this compound is quickly absorbed and metabolized, which can impact its bioavailability.
Result of Action
The action of this compound at the molecular and cellular level results in various effects. For instance, the damage associated with oxidative stress induced by T-2 and its metabolites, including this compound, is relieved by the antioxidant enzymes system on HepG2 cells . This suggests that this compound can modulate the antioxidant defense system of cells, influencing their ability to counteract oxidative stress.
Action Environment
The action, efficacy, and stability of this compound can be influenced by various environmental factors. For instance, the gut microbiota plays a crucial role in the metabolism of T-2 toxin to this compound . This suggests that the gut microbiota can influence the toxicity and efficacy of this compound. Furthermore, the presence of certain compounds, such as NAC and BSO, can modulate the effects of this compound on the antioxidant defense system .
Biochemical Analysis
Biochemical Properties
T-2 triol plays a significant role in biochemical reactions, particularly in the inhibition of protein synthesis. It interacts with various enzymes, proteins, and other biomolecules. One of the primary interactions is with peptidyl-transferase, where this compound binds and inactivates its activity at the transcription site, leading to the inhibition of protein synthesis . Additionally, this compound induces oxidative stress by decreasing glutathione levels and altering the activities of antioxidant enzymes such as glutathione peroxidase (GPx), glutathione S-transferase (GST), and catalase (CAT) .
Cellular Effects
This compound exerts various effects on different types of cells and cellular processes. It induces cell death in primary porcine Leydig cells and affects the antioxidant defense system in HepG2 cells by decreasing glutathione levels and altering the activities of related enzymes . This compound also influences cell signaling pathways, including the JAK/STAT and MAPK pathways, leading to immunotoxicity and apoptosis . Furthermore, it impacts gene expression and cellular metabolism by inducing oxidative stress and promoting apoptosis .
Molecular Mechanism
The molecular mechanism of this compound involves several key processes. It binds to and inactivates peptidyl-transferase activity at the transcription site, resulting in the inhibition of protein synthesis . This compound also induces oxidative stress by decreasing glutathione levels and altering the activities of antioxidant enzymes . Additionally, it affects cell signaling pathways, including the JAK/STAT and MAPK pathways, leading to immunotoxicity and apoptosis . These interactions at the molecular level contribute to the overall toxic effects of this compound.
Temporal Effects in Laboratory Settings
In laboratory settings, the effects of this compound change over time. This compound is rapidly metabolized, and its main metabolites include HT-2, Neosolaniol (Neo), this compound, and T-2 tetraol . The stability and degradation of this compound can influence its long-term effects on cellular function. Studies have shown that this compound induces oxidative stress and alters the activities of antioxidant enzymes over time . These temporal changes are important for understanding the stability and long-term impact of this compound in laboratory settings.
Dosage Effects in Animal Models
The effects of this compound vary with different dosages in animal models. At low doses, this compound can enhance the resistance of animals to pathogens, while at high doses, it can significantly reduce immunity . High doses of this compound can also lead to toxic effects such as vomiting, feed refusal, stomach necrosis, and skin irritation . Understanding the dosage effects of this compound is crucial for assessing its safety and potential adverse effects in animal models.
Metabolic Pathways
This compound is involved in several metabolic pathways, including hydrolysis, hydroxylation, de-epoxidation, and conjugation . The enzymes CYP3A4 and carboxylesterase contribute to the metabolism of this compound, with 3′-hydroxy-T-2 toxin and HT-2 toxin as the primary products . These metabolic pathways play a crucial role in the detoxification and elimination of this compound from the body.
Transport and Distribution
This compound is transported and distributed within cells and tissues through various mechanisms. It is rapidly absorbed from the alimentary tract or through respiratory mucosal membranes and transported to the liver, which is the primary organ responsible for its metabolism . This compound can also be distributed to other organs and tissues, where it exerts its toxic effects. The transport and distribution of this compound are important for understanding its overall impact on the body.
Subcellular Localization
The subcellular localization of this compound plays a significant role in its activity and function. This compound can localize to various cellular compartments, including the cytoplasm and nucleus. It affects the activity of antioxidant enzymes and induces oxidative stress in these compartments . Understanding the subcellular localization of this compound is crucial for elucidating its mechanism of action and overall impact on cellular function.
Preparation Methods
- T-2 Triol is produced through the metabolic transformation of T-2 toxin.
- Industrial production methods are not commonly reported, as it is primarily a metabolite.
Chemical Reactions Analysis
- T-2 Triol can undergo various reactions, including oxidation, reduction, and substitution.
- Common reagents and conditions depend on the specific reaction.
- Major products formed during these reactions are not extensively documented.
Scientific Research Applications
- T-2 Triol has applications in:
Chemistry: Limited studies, but its role as a metabolite is significant.
Biology: Research on its effects in cellular systems.
Medicine: Potential therapeutic implications.
Industry: Minimal industrial applications reported.
Comparison with Similar Compounds
- T-2 Triol’s uniqueness lies in its origin as a metabolite of T-2 toxin.
- Similar compounds include T-2 toxin itself and other trichothecenes.
Biological Activity
T-2 triol, a metabolite of the T-2 toxin produced by the fungus Fusarium sporotrichioides, is recognized for its significant biological activity, particularly its toxicity and effects on various biological systems. This article synthesizes current research findings on this compound, focusing on its biological activity, metabolic pathways, and implications for health in humans and animals.
Overview of this compound
This compound is formed through the hydroxylation of T-2 toxin and is part of a broader group of trichothecene mycotoxins. These compounds are known for their potent cytotoxic and immunotoxic effects across different species. The primary metabolic pathways for T-2 include hydrolysis, hydroxylation, and glucuronidation, leading to various metabolites such as HT-2 toxin and neosolaniol (NEO) .
Biological Activity
Cytotoxic Effects:
this compound exhibits significant cytotoxicity primarily through the inhibition of protein synthesis. This effect is attributed to its interaction with the 60S ribosomal subunit, disrupting polypeptide chain initiation . The most affected tissues are those with high proliferation rates, including the gastrointestinal tract, skin, and bone marrow.
Immunotoxicity:
Research indicates that this compound can induce immunosuppression. For instance, studies have shown decreased leukocyte counts and increased susceptibility to infections in animals exposed to T-2 toxins . In a study involving rabbits, prolonged exposure resulted in liver cell damage and altered serum enzyme levels, highlighting its potential to affect immune function .
Genotoxic Effects:
this compound has been linked to genotoxicity as well. It can cause DNA damage through oxidative stress mechanisms, leading to disturbances in cell signaling pathways . This genotoxic potential raises concerns regarding long-term exposure in agricultural settings where these mycotoxins are prevalent.
Case Studies
-
Poultry Studies:
A notable case study reported on laying hens exposed to T-2 toxin showed a 22% reduction in egg production and increased incidence of oral lesions . These findings underscore the economic impact of this compound on poultry production. -
Rodent Models:
In Wistar rats exposed to varying doses of T-2 toxin, significant immunosuppressive effects were observed, including alterations in both humoral and cell-mediated immune responses . Additionally, cardiac tissue injury was documented following chronic exposure . -
Human Exposure:
Human biomonitoring studies have detected this compound and its glucoside forms in human samples, indicating potential dietary exposure risks from contaminated grains . The metabolic profile of these compounds remains an area requiring further investigation to understand their implications fully.
Metabolic Pathways
The metabolism of T-2 toxin involves several key pathways:
Metabolite | Pathway | Biological Significance |
---|---|---|
HT-2 | Hydrolysis | Less toxic than T-2; serves as a biomarker for exposure |
3′-OH-T-2 | Hydroxylation | Main product in certain species; potential biomarker |
3′-OH-HT-2 | Hydroxylation | Predominant in goats and swine; indicates species-specific metabolism |
T-2-3-glucuronide | Glucuronidation | Conjugated form; may influence detoxification processes |
Properties
IUPAC Name |
[10,11-dihydroxy-2-(hydroxymethyl)-1,5-dimethylspiro[8-oxatricyclo[7.2.1.02,7]dodec-5-ene-12,2'-oxirane]-4-yl] 3-methylbutanoate | |
---|---|---|
Details | Computed by Lexichem TK 2.7.0 (PubChem release 2021.05.07) | |
Source | PubChem | |
URL | https://pubchem.ncbi.nlm.nih.gov | |
Description | Data deposited in or computed by PubChem | |
InChI |
InChI=1S/C20H30O7/c1-10(2)5-14(22)26-12-7-19(8-21)13(6-11(12)3)27-17-15(23)16(24)18(19,4)20(17)9-25-20/h6,10,12-13,15-17,21,23-24H,5,7-9H2,1-4H3 | |
Details | Computed by InChI 1.0.6 (PubChem release 2021.05.07) | |
Source | PubChem | |
URL | https://pubchem.ncbi.nlm.nih.gov | |
Description | Data deposited in or computed by PubChem | |
InChI Key |
DDAUKBBLCGQHIP-UHFFFAOYSA-N | |
Details | Computed by InChI 1.0.6 (PubChem release 2021.05.07) | |
Source | PubChem | |
URL | https://pubchem.ncbi.nlm.nih.gov | |
Description | Data deposited in or computed by PubChem | |
Canonical SMILES |
CC1=CC2C(CC1OC(=O)CC(C)C)(C3(C(C(C(C34CO4)O2)O)O)C)CO | |
Details | Computed by OEChem 2.3.0 (PubChem release 2021.05.07) | |
Source | PubChem | |
URL | https://pubchem.ncbi.nlm.nih.gov | |
Description | Data deposited in or computed by PubChem | |
Molecular Formula |
C20H30O7 | |
Details | Computed by PubChem 2.1 (PubChem release 2021.05.07) | |
Source | PubChem | |
URL | https://pubchem.ncbi.nlm.nih.gov | |
Description | Data deposited in or computed by PubChem | |
Molecular Weight |
382.4 g/mol | |
Details | Computed by PubChem 2.1 (PubChem release 2021.05.07) | |
Source | PubChem | |
URL | https://pubchem.ncbi.nlm.nih.gov | |
Description | Data deposited in or computed by PubChem | |
CAS No. |
97373-21-2, 34114-98-2 | |
Record name | [10,11-dihydroxy-2-(hydroxymethyl)-1,5-dimethylspiro[8-oxatricyclo[7.2.1.02,7]dodec-5-ene-12,2'-oxirane]-4-yl] 3-methylbutanoate | |
Source | European Chemicals Agency (ECHA) | |
URL | https://echa.europa.eu/information-on-chemicals | |
Description | The European Chemicals Agency (ECHA) is an agency of the European Union which is the driving force among regulatory authorities in implementing the EU's groundbreaking chemicals legislation for the benefit of human health and the environment as well as for innovation and competitiveness. | |
Explanation | Use of the information, documents and data from the ECHA website is subject to the terms and conditions of this Legal Notice, and subject to other binding limitations provided for under applicable law, the information, documents and data made available on the ECHA website may be reproduced, distributed and/or used, totally or in part, for non-commercial purposes provided that ECHA is acknowledged as the source: "Source: European Chemicals Agency, http://echa.europa.eu/". Such acknowledgement must be included in each copy of the material. ECHA permits and encourages organisations and individuals to create links to the ECHA website under the following cumulative conditions: Links can only be made to webpages that provide a link to the Legal Notice page. | |
Record name | T2 Triol | |
Source | Human Metabolome Database (HMDB) | |
URL | http://www.hmdb.ca/metabolites/HMDB0035396 | |
Description | The Human Metabolome Database (HMDB) is a freely available electronic database containing detailed information about small molecule metabolites found in the human body. | |
Explanation | HMDB is offered to the public as a freely available resource. Use and re-distribution of the data, in whole or in part, for commercial purposes requires explicit permission of the authors and explicit acknowledgment of the source material (HMDB) and the original publication (see the HMDB citing page). We ask that users who download significant portions of the database cite the HMDB paper in any resulting publications. | |
Retrosynthesis Analysis
AI-Powered Synthesis Planning: Our tool employs the Template_relevance Pistachio, Template_relevance Bkms_metabolic, Template_relevance Pistachio_ringbreaker, Template_relevance Reaxys, Template_relevance Reaxys_biocatalysis model, leveraging a vast database of chemical reactions to predict feasible synthetic routes.
One-Step Synthesis Focus: Specifically designed for one-step synthesis, it provides concise and direct routes for your target compounds, streamlining the synthesis process.
Accurate Predictions: Utilizing the extensive PISTACHIO, BKMS_METABOLIC, PISTACHIO_RINGBREAKER, REAXYS, REAXYS_BIOCATALYSIS database, our tool offers high-accuracy predictions, reflecting the latest in chemical research and data.
Strategy Settings
Precursor scoring | Relevance Heuristic |
---|---|
Min. plausibility | 0.01 |
Model | Template_relevance |
Template Set | Pistachio/Bkms_metabolic/Pistachio_ringbreaker/Reaxys/Reaxys_biocatalysis |
Top-N result to add to graph | 6 |
Feasible Synthetic Routes
Q1: How does T-2 triol compare in toxicity to its parent compound, T-2 toxin?
A1: this compound consistently demonstrates lower toxicity compared to T-2 toxin. Studies using human lymphocytes found that this compound required significantly higher concentrations to inhibit cell proliferation compared to T-2 toxin. [] Similar results were obtained in studies with brine shrimp and protozoan Tetrahymena pyriformis, where this compound exhibited lower toxicity than T-2 toxin. [, ] This suggests that the metabolic conversion of T-2 toxin to this compound generally represents a detoxification step.
Q2: What are the main metabolic pathways of T-2 toxin involving this compound in animals?
A2: T-2 toxin is primarily metabolized through hydrolysis of its ester side chains. This process occurs sequentially, with HT-2 toxin as the initial metabolite. HT-2 toxin can be further hydrolyzed to form this compound. [, ] This pathway ultimately leads to the formation of T-2 tetraol, the least toxic metabolite in the series.
Q3: Can this compound be further metabolized, and what are the implications for toxicity?
A3: Yes, research indicates that some bacterial strains can further degrade this compound. One study identified a Curtobacterium sp. strain capable of transforming T-2 toxin to HT-2 toxin and subsequently to this compound, without the formation of other intermediates like neosolaniol. [] Further investigation revealed that this bacterium could completely degrade this compound, removing it from the culture medium. [] Such microbial degradation pathways are important for detoxification and reducing the levels of this compound in contaminated environments.
Q4: How does the metabolism of T-2 toxin differ between animals and humans?
A4: While the primary metabolic route of T-2 toxin, involving the formation of HT-2 toxin and this compound, is conserved across species, some differences exist. In humans, HT-2 toxin appears to be the main circulating metabolite. [] In contrast, animals tend to further metabolize HT-2 toxin into 3'-hydroxy-HT-2 toxin and this compound, ultimately leading to T-2 tetraol. [] Notably, de-epoxidation, a key detoxification mechanism observed in animals, has not been reported in humans. []
Q5: What is the significance of identifying this compound in biological samples?
A5: The presence of this compound, even at low concentrations, can indicate prior exposure to T-2 toxin. Research has successfully identified this compound in chicken excreta and tissues, confirming its presence as a metabolite. [] The detection of this compound, along with other T-2 metabolites, can help assess the extent of exposure to this mycotoxin and highlight potential risks associated with contaminated feed.
Q6: What analytical techniques are commonly employed to detect and quantify this compound?
A6: Gas chromatography coupled with mass spectrometry (GC/MS) is widely used for the identification and quantification of this compound in various matrices. [, , ] This method provides high sensitivity and selectivity for analyzing complex mixtures of trichothecenes. Liquid chromatography coupled with tandem mass spectrometry (LC-MS/MS) is another powerful tool for analyzing this compound and other trichothecenes simultaneously. [, , , ]
Q7: Have any studies investigated the presence of masked this compound in feed and food?
A7: Yes, research has explored the potential for masked mycotoxins, including this compound, in cereal straw. [] While the study did not find evidence for the release of masked this compound using chemical and enzymatic hydrolysis methods, it highlighted the need for further investigation using more advanced techniques to fully understand the occurrence and significance of masked mycotoxins. []
Q8: In what types of food and feed samples has this compound been detected?
A8: this compound, alongside other T-2 toxin metabolites, has been found in various agricultural commodities, including oats, maize, and wheat. [, , , ] Its presence in these staple grains raises concerns about potential exposure through the food chain, especially for livestock consuming contaminated feed.
Disclaimer and Information on In-Vitro Research Products
Please be aware that all articles and product information presented on BenchChem are intended solely for informational purposes. The products available for purchase on BenchChem are specifically designed for in-vitro studies, which are conducted outside of living organisms. In-vitro studies, derived from the Latin term "in glass," involve experiments performed in controlled laboratory settings using cells or tissues. It is important to note that these products are not categorized as medicines or drugs, and they have not received approval from the FDA for the prevention, treatment, or cure of any medical condition, ailment, or disease. We must emphasize that any form of bodily introduction of these products into humans or animals is strictly prohibited by law. It is essential to adhere to these guidelines to ensure compliance with legal and ethical standards in research and experimentation.