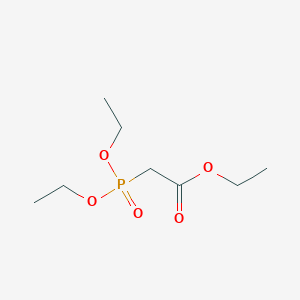
Triethyl phosphonoacetate
Overview
Description
Triethyl phosphonoacetate is an organophosphorus compound with the chemical formula C8H17O5P . It is a colorless to light yellow liquid that is primarily used as a reagent in organic synthesis, particularly in the Horner-Wadsworth-Emmons reaction. This reaction is a modification of the Wittig reaction and is used to form carbon-carbon double bonds with high regioselectivity .
Mechanism of Action
Target of Action
Triethyl phosphonoacetate is primarily used as a reagent in organic synthesis . Its primary target is the carbonyl group in aldehydes and ketones . The carbonyl group plays a crucial role in many biochemical reactions, and its modification can lead to significant changes in the properties of the molecule.
Mode of Action
This compound interacts with its targets through the Horner-Wadsworth-Emmons (HWE) reaction or the Horner-Emmons modification . It can be added dropwise to a sodium methoxide solution to prepare a phosphonate anion . It has an acidic proton that can easily be abstracted by a weak base . When used in an HWE reaction with a carbonyl, the resulting alkene formed is usually the E alkene, and is generated with excellent regioselectivity .
Biochemical Pathways
The primary biochemical pathway affected by this compound is the formation of alkenes through the HWE reaction . This reaction is a method for the formation of carbon-carbon double bonds (alkenes) by the reaction of an aldehyde or ketone with a phosphonate anion . The downstream effects include the synthesis of various organic compounds, including aldehydes .
Result of Action
The primary result of this compound’s action is the formation of E alkenes with excellent regioselectivity . This allows for the synthesis of a wide range of organic compounds, including aldehydes .
Biochemical Analysis
Biochemical Properties
Triethyl phosphonoacetate plays a crucial role in biochemical reactions, particularly in the formation of alkenes through the Horner-Wadsworth-Emmons reaction. This reaction involves the interaction of this compound with carbonyl compounds, leading to the formation of E-alkenes with excellent regioselectivity . The compound can be deprotonated by a weak base to form a phosphonate anion, which then participates in the reaction. This compound interacts with enzymes and proteins involved in metabolic pathways, although specific interactions with biomolecules in biological systems are less documented.
Molecular Mechanism
At the molecular level, this compound exerts its effects through the formation of a phosphonate anion upon deprotonation by a weak base . This anion then reacts with carbonyl compounds to form alkenes. The stereochemistry of the reaction is controlled by steric approach control, favoring the antiperiplanar approach of the carbanion to the carbonyl carbon . This mechanism is similar to the Wittig reaction, with the phosphonate anion acting as the nucleophile.
Temporal Effects in Laboratory Settings
In laboratory settings, the stability and degradation of this compound are important considerations. The compound is stable under standard conditions but may degrade over time when exposed to light and moisture
Metabolic Pathways
This compound is involved in metabolic pathways related to the synthesis of alkenes through the Horner-Wadsworth-Emmons reaction The compound interacts with enzymes and cofactors that facilitate the formation of phosphonate anions and their subsequent reactions with carbonyl compounds
Preparation Methods
Synthetic Routes and Reaction Conditions
Triethyl phosphonoacetate can be synthesized through the Arbuzov reaction, which involves the reaction of triethyl phosphite with ethyl bromoacetate. The reaction typically occurs under reflux conditions in the presence of a solvent such as toluene .
Industrial Production Methods
In an industrial setting, the preparation of this compound involves heating a mixture of triethyl phosphite and ethyl bromoacetate in a continuous flow reactor. The reaction is carried out at elevated temperatures and pressures to ensure high yield and purity. The product is then purified through distillation .
Chemical Reactions Analysis
Types of Reactions
Triethyl phosphonoacetate undergoes several types of chemical reactions, including:
Oxidation: It can be oxidized to form phosphonic acids.
Reduction: It can be reduced to form phosphonates.
Substitution: It can undergo nucleophilic substitution reactions to form various derivatives.
Common Reagents and Conditions
Oxidation: Common oxidizing agents include hydrogen peroxide and potassium permanganate.
Reduction: Reducing agents such as lithium aluminum hydride are used.
Substitution: Bases like sodium methoxide are commonly used to generate the phosphonate anion.
Major Products Formed
Oxidation: Phosphonic acids.
Reduction: Phosphonates.
Substitution: Various phosphonate derivatives.
Scientific Research Applications
Triethyl phosphonoacetate has a wide range of applications in scientific research:
Chemistry: It is used in the synthesis of alkenes through the Horner-Wadsworth-Emmons reaction.
Medicine: It is used in the synthesis of antiviral and anticancer drugs.
Industry: It is used in the production of polymers, adhesives, and coatings.
Comparison with Similar Compounds
Similar Compounds
- Diethyl phosphonoacetate
- Trimethyl phosphonoacetate
- Triethyl phosphonopropionate
Uniqueness
Triethyl phosphonoacetate is unique due to its high regioselectivity in forming E-alkenes in the Horner-Wadsworth-Emmons reaction. This selectivity is less pronounced with ketones compared to aldehydes, making it particularly useful in the synthesis of complex organic molecules .
Biological Activity
Triethyl phosphonoacetate (TEPA) is an organophosphorus compound that has garnered attention for its diverse biological activities, particularly in synthetic organic chemistry and potential applications in medicinal chemistry. This article explores the biological activity of TEPA, highlighting its synthesis, mechanisms of action, and relevant case studies.
Chemical Structure and Properties
This compound is characterized by the following chemical structure:
- Molecular Formula : CHOP
- IUPAC Name : Ethyl 2-(diethoxyphosphoryl)acetate
- CAS Number : 867-13-0
The compound is a colorless liquid with a density of 1.13 g/mL and a boiling point of 142-145 °C at 9 mmHg .
Synthesis and Applications
TEPA is primarily used as a precursor in the synthesis of various biologically active compounds. It plays a significant role in the Horner-Wadsworth-Emmons reaction, which is crucial for forming carbon-carbon double bonds in organic synthesis . Additionally, TEPA has been utilized in the synthesis of heterocyclic compounds with potential pharmacological properties .
- Anticancer Activity : TEPA has shown promise in the synthesis of compounds that exhibit anticancer properties. For instance, derivatives synthesized from TEPA have been evaluated for their efficacy against cancer cell lines, demonstrating significant cytotoxic effects .
- Cardiotonic Effects : Research indicates that TEPA-derived compounds can enhance cardiac contractility. In studies involving anesthetized dogs, certain derivatives produced greater increases in cardiac contractility compared to other analogues .
- Decontamination Efficacy : TEPA has been studied for its potential to decontaminate skin exposed to organophosphorus compounds (OPCs), including chemical warfare agents. In vitro studies have shown that TEPA can effectively degrade these toxic agents when combined with reactive skin decontamination lotions (RSDL) .
- Toxicity and Safety : While TEPA is useful in various applications, it also poses risks associated with organophosphorus compounds, such as potential neurotoxicity and skin irritation . Safety measures are essential when handling this compound.
Case Study 1: Synthesis of Cardiotonic Agents
A series of 6-(N-linked, five-membered heteroaryl)-2(1H)-quinolinones were synthesized using the ylide derived from TEPA. These compounds were evaluated for their cardiotonic activity, revealing promising results that warrant further investigation into their therapeutic applications .
Case Study 2: Decontamination Studies
In a study assessing the efficacy of RSDL on skin contaminated with OPCs, TEPA was compared to VX (a nerve agent). The findings indicated that early decontamination was crucial for effectiveness, with TEPA showing significant degradation capabilities under specific conditions .
Data Table: Biological Activities of this compound Derivatives
Properties
IUPAC Name |
ethyl 2-diethoxyphosphorylacetate | |
---|---|---|
Source | PubChem | |
URL | https://pubchem.ncbi.nlm.nih.gov | |
Description | Data deposited in or computed by PubChem | |
InChI |
InChI=1S/C8H17O5P/c1-4-11-8(9)7-14(10,12-5-2)13-6-3/h4-7H2,1-3H3 | |
Source | PubChem | |
URL | https://pubchem.ncbi.nlm.nih.gov | |
Description | Data deposited in or computed by PubChem | |
InChI Key |
GGUBFICZYGKNTD-UHFFFAOYSA-N | |
Source | PubChem | |
URL | https://pubchem.ncbi.nlm.nih.gov | |
Description | Data deposited in or computed by PubChem | |
Canonical SMILES |
CCOC(=O)CP(=O)(OCC)OCC | |
Source | PubChem | |
URL | https://pubchem.ncbi.nlm.nih.gov | |
Description | Data deposited in or computed by PubChem | |
Molecular Formula |
C8H17O5P | |
Source | PubChem | |
URL | https://pubchem.ncbi.nlm.nih.gov | |
Description | Data deposited in or computed by PubChem | |
DSSTOX Substance ID |
DTXSID4041573 | |
Record name | Triethyl phosphonoacetate | |
Source | EPA DSSTox | |
URL | https://comptox.epa.gov/dashboard/DTXSID4041573 | |
Description | DSSTox provides a high quality public chemistry resource for supporting improved predictive toxicology. | |
Molecular Weight |
224.19 g/mol | |
Source | PubChem | |
URL | https://pubchem.ncbi.nlm.nih.gov | |
Description | Data deposited in or computed by PubChem | |
Physical Description |
Clear light yellow liquid; [Acros Organics MSDS] | |
Record name | Triethyl phosphonoacetate | |
Source | Haz-Map, Information on Hazardous Chemicals and Occupational Diseases | |
URL | https://haz-map.com/Agents/19899 | |
Description | Haz-Map® is an occupational health database designed for health and safety professionals and for consumers seeking information about the adverse effects of workplace exposures to chemical and biological agents. | |
Explanation | Copyright (c) 2022 Haz-Map(R). All rights reserved. Unless otherwise indicated, all materials from Haz-Map are copyrighted by Haz-Map(R). No part of these materials, either text or image may be used for any purpose other than for personal use. Therefore, reproduction, modification, storage in a retrieval system or retransmission, in any form or by any means, electronic, mechanical or otherwise, for reasons other than personal use, is strictly prohibited without prior written permission. | |
CAS No. |
867-13-0 | |
Record name | Triethyl phosphonoacetate | |
Source | CAS Common Chemistry | |
URL | https://commonchemistry.cas.org/detail?cas_rn=867-13-0 | |
Description | CAS Common Chemistry is an open community resource for accessing chemical information. Nearly 500,000 chemical substances from CAS REGISTRY cover areas of community interest, including common and frequently regulated chemicals, and those relevant to high school and undergraduate chemistry classes. This chemical information, curated by our expert scientists, is provided in alignment with our mission as a division of the American Chemical Society. | |
Explanation | The data from CAS Common Chemistry is provided under a CC-BY-NC 4.0 license, unless otherwise stated. | |
Record name | Triethyl phosphonoacetate | |
Source | ChemIDplus | |
URL | https://pubchem.ncbi.nlm.nih.gov/substance/?source=chemidplus&sourceid=0000867130 | |
Description | ChemIDplus is a free, web search system that provides access to the structure and nomenclature authority files used for the identification of chemical substances cited in National Library of Medicine (NLM) databases, including the TOXNET system. | |
Record name | Triethyl phosphonoacetate | |
Source | DTP/NCI | |
URL | https://dtp.cancer.gov/dtpstandard/servlet/dwindex?searchtype=NSC&outputformat=html&searchlist=16128 | |
Description | The NCI Development Therapeutics Program (DTP) provides services and resources to the academic and private-sector research communities worldwide to facilitate the discovery and development of new cancer therapeutic agents. | |
Explanation | Unless otherwise indicated, all text within NCI products is free of copyright and may be reused without our permission. Credit the National Cancer Institute as the source. | |
Record name | Triethyl phosphonoacetate | |
Source | DTP/NCI | |
URL | https://dtp.cancer.gov/dtpstandard/servlet/dwindex?searchtype=NSC&outputformat=html&searchlist=13898 | |
Description | The NCI Development Therapeutics Program (DTP) provides services and resources to the academic and private-sector research communities worldwide to facilitate the discovery and development of new cancer therapeutic agents. | |
Explanation | Unless otherwise indicated, all text within NCI products is free of copyright and may be reused without our permission. Credit the National Cancer Institute as the source. | |
Record name | Acetic acid, 2-(diethoxyphosphinyl)-, ethyl ester | |
Source | EPA Chemicals under the TSCA | |
URL | https://www.epa.gov/chemicals-under-tsca | |
Description | EPA Chemicals under the Toxic Substances Control Act (TSCA) collection contains information on chemicals and their regulations under TSCA, including non-confidential content from the TSCA Chemical Substance Inventory and Chemical Data Reporting. | |
Record name | Triethyl phosphonoacetate | |
Source | EPA DSSTox | |
URL | https://comptox.epa.gov/dashboard/DTXSID4041573 | |
Description | DSSTox provides a high quality public chemistry resource for supporting improved predictive toxicology. | |
Record name | Triethyl phosphonoacetate | |
Source | European Chemicals Agency (ECHA) | |
URL | https://echa.europa.eu/substance-information/-/substanceinfo/100.011.598 | |
Description | The European Chemicals Agency (ECHA) is an agency of the European Union which is the driving force among regulatory authorities in implementing the EU's groundbreaking chemicals legislation for the benefit of human health and the environment as well as for innovation and competitiveness. | |
Explanation | Use of the information, documents and data from the ECHA website is subject to the terms and conditions of this Legal Notice, and subject to other binding limitations provided for under applicable law, the information, documents and data made available on the ECHA website may be reproduced, distributed and/or used, totally or in part, for non-commercial purposes provided that ECHA is acknowledged as the source: "Source: European Chemicals Agency, http://echa.europa.eu/". Such acknowledgement must be included in each copy of the material. ECHA permits and encourages organisations and individuals to create links to the ECHA website under the following cumulative conditions: Links can only be made to webpages that provide a link to the Legal Notice page. | |
Record name | TRIETHYL PHOSPHONOACETATE | |
Source | FDA Global Substance Registration System (GSRS) | |
URL | https://gsrs.ncats.nih.gov/ginas/app/beta/substances/022826171T | |
Description | The FDA Global Substance Registration System (GSRS) enables the efficient and accurate exchange of information on what substances are in regulated products. Instead of relying on names, which vary across regulatory domains, countries, and regions, the GSRS knowledge base makes it possible for substances to be defined by standardized, scientific descriptions. | |
Explanation | Unless otherwise noted, the contents of the FDA website (www.fda.gov), both text and graphics, are not copyrighted. They are in the public domain and may be republished, reprinted and otherwise used freely by anyone without the need to obtain permission from FDA. Credit to the U.S. Food and Drug Administration as the source is appreciated but not required. | |
Synthesis routes and methods
Procedure details
Retrosynthesis Analysis
AI-Powered Synthesis Planning: Our tool employs the Template_relevance Pistachio, Template_relevance Bkms_metabolic, Template_relevance Pistachio_ringbreaker, Template_relevance Reaxys, Template_relevance Reaxys_biocatalysis model, leveraging a vast database of chemical reactions to predict feasible synthetic routes.
One-Step Synthesis Focus: Specifically designed for one-step synthesis, it provides concise and direct routes for your target compounds, streamlining the synthesis process.
Accurate Predictions: Utilizing the extensive PISTACHIO, BKMS_METABOLIC, PISTACHIO_RINGBREAKER, REAXYS, REAXYS_BIOCATALYSIS database, our tool offers high-accuracy predictions, reflecting the latest in chemical research and data.
Strategy Settings
Precursor scoring | Relevance Heuristic |
---|---|
Min. plausibility | 0.01 |
Model | Template_relevance |
Template Set | Pistachio/Bkms_metabolic/Pistachio_ringbreaker/Reaxys/Reaxys_biocatalysis |
Top-N result to add to graph | 6 |
Feasible Synthetic Routes
Q1: What is the molecular formula and weight of Triethyl phosphonoacetate?
A1: this compound has the molecular formula C8H17O5P and a molecular weight of 224.19 g/mol.
Q2: Is there any spectroscopic data available for this compound?
A2: Yes, studies have utilized various spectroscopic techniques for characterization. For example, one study employed 31P NMR to analyze the anionic species formed from this compound under specific reaction conditions . Another research investigated UV Raman cross sections of this compound, providing valuable data for its detection and analysis .
Q3: What is the primary use of this compound in organic synthesis?
A3: this compound is a crucial reagent in the Horner-Wadsworth-Emmons (HWE) reaction. This reaction is a widely used method for synthesizing α,β-unsaturated esters, ketones, and other conjugated systems .
Q4: Can you explain the role of this compound in the HWE reaction?
A4: In the HWE reaction, this compound acts as a nucleophile. It forms an enolate in the presence of a base, which then attacks the carbonyl group of an aldehyde or ketone. This initiates a series of steps, ultimately leading to the formation of an alkene and the expulsion of a phosphate group .
Q5: What is the stereochemical outcome of the HWE reaction using this compound?
A5: Generally, this compound in the HWE reaction predominantly yields E-olefins. This selectivity arises from the preferential formation of the thermodynamically favored threo adduct during the reaction .
Q6: Are there exceptions to this E-selectivity in the HWE reaction with this compound?
A6: Yes, research has shown that exceptions exist. For example, the reaction of this compound with benzyl 2-pyridyl ketone under specific conditions (t-BuOK as a base) produced a 1:1 mixture of E and Z olefins . This finding highlights the influence of substrate structure and reaction conditions on the stereochemical outcome.
Q7: What are some specific applications of this compound in synthesis?
A7: this compound finds use in diverse syntheses, including:* β-Keto Phosphonates: A practical synthesis of β-keto phosphonates utilizes this compound, reacting it with carboxylic acid chlorides followed by decarboxylation , .* 3(2H)-Pyridazinones: this compound reacts with phenylhydrazones of 1,2-dicarbonyl compounds to synthesize various 5,6-disubstituted-2-phenyl-3(2H)-pyridazinones .* 1,3,4-oxadiazoles: Phosphonomethylhydrazide, derived from this compound, reacts with N-acylated imidates to yield 5-aryl (or 5-benzyl)-2-[(1-diethoxyphosphonyl)methyl]-1,3,4-oxadiazoles .* Cyclopropanes: this compound plays a key role in the Wadsworth-Emmons cyclopropanation, a method for synthesizing optically pure, multi-substituted cyclopropanes with high trans-diastereoselectivity .
Q8: Has the stability of this compound been investigated under extreme conditions?
A8: Yes, researchers have explored the behavior of this compound under high temperatures and pressures. A study investigated its aquathermolysis, examining its degradation in superheated water under sub- and supercritical conditions, demonstrating its potential for detoxification of specific compounds .
Q9: Are there alternative methods for conducting reactions with this compound besides traditional solvent-based approaches?
A9: Yes, researchers have explored alternative reaction media. For instance, one study successfully employed the ionic liquid 1-butyl-3-methylimidazolium hexafluorophosphate ([bmim][PF6]) as a solvent for reactions involving this compound and aldehydes, offering potential advantages in terms of reaction rates and selectivity .
Q10: Have solvent-free conditions been explored for reactions involving this compound?
A10: Yes, research has demonstrated the effectiveness of solvent-free conditions in the presence of specific catalysts. For example, the Horner-Wadsworth-Emmons reaction of this compound with aldehydes proceeded with high E-selectivity when catalyzed by DBU (1,8-Diazabicyclo[5.4.0]undec-7-ene) in the presence of K2CO3 under solvent-free conditions .
Disclaimer and Information on In-Vitro Research Products
Please be aware that all articles and product information presented on BenchChem are intended solely for informational purposes. The products available for purchase on BenchChem are specifically designed for in-vitro studies, which are conducted outside of living organisms. In-vitro studies, derived from the Latin term "in glass," involve experiments performed in controlled laboratory settings using cells or tissues. It is important to note that these products are not categorized as medicines or drugs, and they have not received approval from the FDA for the prevention, treatment, or cure of any medical condition, ailment, or disease. We must emphasize that any form of bodily introduction of these products into humans or animals is strictly prohibited by law. It is essential to adhere to these guidelines to ensure compliance with legal and ethical standards in research and experimentation.