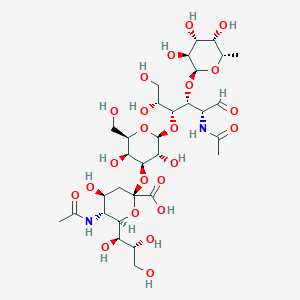
Sialyl-Lewis X
Overview
Description
2,3-Diaminophenazinium chloride, commonly referred to as DAPH, is a phenazine derivative. Phenazine compounds are known for their diverse biological activities and are often used in medicinal chemistry and materials science. DAPH is particularly noted for its potential therapeutic properties, including antibacterial and antiviral activities .
Biochemical Analysis
Biochemical Properties
Sialyl-Lewis X is involved in several biochemical reactions, primarily through its interactions with selectins, a family of cell adhesion molecules. It serves as a ligand for E-selectin, P-selectin, and L-selectin, facilitating the adhesion of leukocytes to endothelial cells during the inflammatory response . This interaction is mediated by the binding of this compound to the lectin domain of selectins, which is crucial for the rolling and tethering of leukocytes on the vascular endothelium . Additionally, this compound interacts with various glycosyltransferases and sialyltransferases, which are responsible for its biosynthesis and modification .
Cellular Effects
This compound influences various cellular processes, including cell signaling, gene expression, and cellular metabolism. In immune cells, it plays a pivotal role in the trafficking and homing of leukocytes to sites of inflammation . The binding of this compound to selectins triggers intracellular signaling pathways that enhance the expression of adhesion molecules and cytokines, promoting the inflammatory response . In cancer cells, overexpression of this compound is associated with increased metastatic potential, as it facilitates the adhesion and extravasation of tumor cells into distant tissues .
Molecular Mechanism
The molecular mechanism of this compound involves its specific binding interactions with selectins and other biomolecules. The tetrasaccharide structure of this compound allows it to fit into the binding pocket of selectins, forming multiple hydrogen bonds and van der Waals interactions . This binding induces conformational changes in the selectin molecules, enhancing their affinity for leukocytes and tumor cells . Additionally, this compound can modulate the activity of glycosyltransferases and sialyltransferases, influencing the glycosylation patterns of other glycoproteins and glycolipids .
Temporal Effects in Laboratory Settings
In laboratory settings, the effects of this compound can vary over time due to its stability and degradation. Studies have shown that this compound is relatively stable under physiological conditions, but its activity can be affected by enzymatic degradation and chemical modifications . Long-term exposure to this compound in in vitro and in vivo studies has demonstrated sustained effects on leukocyte adhesion and tumor cell metastasis, highlighting its potential as a therapeutic target .
Dosage Effects in Animal Models
The effects of this compound in animal models are dose-dependent. Low doses of this compound have been shown to enhance leukocyte adhesion and migration, while high doses can lead to adverse effects such as excessive inflammation and tissue damage . In cancer models, varying doses of this compound have been used to study its impact on tumor growth and metastasis, with higher doses generally correlating with increased metastatic potential .
Metabolic Pathways
This compound is involved in several metabolic pathways, including those related to glycosylation and sialylation. It is synthesized by the sequential action of glycosyltransferases and sialyltransferases, which add specific sugar residues to the growing oligosaccharide chain . This compound can also influence metabolic flux and metabolite levels by modulating the activity of these enzymes and altering the glycosylation patterns of other biomolecules .
Transport and Distribution
Within cells and tissues, this compound is transported and distributed through interactions with specific transporters and binding proteins. It is primarily localized on the cell surface, where it can interact with selectins and other cell adhesion molecules . The distribution of this compound can be influenced by its biosynthesis and degradation, as well as by the presence of other glycoproteins and glycolipids that share similar glycosylation patterns .
Subcellular Localization
This compound is predominantly localized on the plasma membrane, where it plays a key role in cell-cell interactions and signaling . It can also be found in the Golgi apparatus, where it is synthesized and modified by glycosyltransferases and sialyltransferases . The subcellular localization of this compound is regulated by targeting signals and post-translational modifications that direct it to specific compartments and organelles .
Preparation Methods
Synthetic Routes and Reaction Conditions
DAPH can be synthesized through the direct catalytic oxidation of o-phenylenediamine with an iron(III) complex, specifically [Fe(1,10-phenanthroline)2Cl2]NO3, in ethanol under aerobic conditions . The reaction involves the following steps:
- Dissolve o-phenylenediamine in ethanol.
- Add the iron(III) complex to the solution.
- Allow the reaction to proceed under aerobic conditions.
- Isolate the product through crystallization.
The crystal structure and molecular complexity of DAPH are confirmed using various spectroscopic methods and single-crystal X-ray structural analysis .
Industrial Production Methods
While specific industrial production methods for DAPH are not extensively documented, the general approach involves scaling up the laboratory synthesis process. This includes optimizing reaction conditions, such as temperature, pressure, and solvent choice, to ensure high yield and purity. Industrial production may also involve continuous flow reactors to enhance efficiency and scalability.
Chemical Reactions Analysis
Types of Reactions
DAPH undergoes several types of chemical reactions, including:
Oxidation: DAPH can be oxidized to form various phenazine derivatives.
Reduction: Reduction reactions can convert DAPH into its reduced forms, which may exhibit different biological activities.
Substitution: DAPH can participate in substitution reactions, where functional groups on the phenazine ring are replaced with other groups.
Common Reagents and Conditions
Oxidation: Common oxidizing agents include hydrogen peroxide and potassium permanganate.
Reduction: Reducing agents such as sodium borohydride or lithium aluminum hydride are used.
Substitution: Substitution reactions often involve nucleophiles like amines or thiols under basic conditions.
Major Products
The major products formed from these reactions depend on the specific reagents and conditions used. For example, oxidation of DAPH can yield various phenazine derivatives with potential biological activities .
Scientific Research Applications
DAPH has a wide range of scientific research applications:
Chemistry: DAPH is used as a building block for synthesizing other phenazine derivatives.
Biology: DAPH exhibits antibacterial properties against clinical bacteria such as Streptococcus pneumoniae and Escherichia coli.
Industry: DAPH’s antibacterial properties make it a candidate for use in disinfectants and preservatives.
Mechanism of Action
DAPH exerts its effects through several mechanisms:
Inhibition of Enzymes: DAPH can inhibit enzymes such as BACE1, which is involved in the formation of amyloid-beta plaques in Alzheimer’s disease.
Antioxidant Activity: DAPH activates the Nrf2/HO-1 pathway, which helps reduce oxidative stress and inflammation.
Antibacterial Activity: DAPH disrupts bacterial cell membranes, leading to cell death.
Comparison with Similar Compounds
DAPH can be compared with other phenazine derivatives, such as:
Phenazine-1-carboxylic acid: Known for its antifungal properties.
Pyocyanin: Exhibits antibacterial and cytotoxic activities.
Phenazine-1,6-dicarboxylic acid: Used in the synthesis of various organic compounds.
Uniqueness
DAPH is unique due to its dual antibacterial and antiviral activities, as well as its potential therapeutic applications in neurodegenerative diseases . Its ability to inhibit amyloid-beta fibril formation sets it apart from other phenazine derivatives.
Properties
IUPAC Name |
(2S,4S,5R,6R)-5-acetamido-2-[(2S,3R,4S,5S,6R)-2-[(2R,3R,4R,5R)-5-acetamido-1,2-dihydroxy-6-oxo-4-[(2S,3S,4R,5S,6S)-3,4,5-trihydroxy-6-methyloxan-2-yl]oxyhexan-3-yl]oxy-3,5-dihydroxy-6-(hydroxymethyl)oxan-4-yl]oxy-4-hydroxy-6-[(1R,2R)-1,2,3-trihydroxypropyl]oxane-2-carboxylic acid | |
---|---|---|
Source | PubChem | |
URL | https://pubchem.ncbi.nlm.nih.gov | |
Description | Data deposited in or computed by PubChem | |
InChI |
InChI=1S/C31H52N2O23/c1-9-18(43)21(46)22(47)28(51-9)53-24(12(5-34)32-10(2)38)25(15(42)7-36)54-29-23(48)27(20(45)16(8-37)52-29)56-31(30(49)50)4-13(40)17(33-11(3)39)26(55-31)19(44)14(41)6-35/h5,9,12-29,35-37,40-48H,4,6-8H2,1-3H3,(H,32,38)(H,33,39)(H,49,50)/t9-,12-,13-,14+,15+,16+,17+,18+,19+,20-,21+,22-,23+,24+,25+,26+,27-,28-,29-,31-/m0/s1 | |
Source | PubChem | |
URL | https://pubchem.ncbi.nlm.nih.gov | |
Description | Data deposited in or computed by PubChem | |
InChI Key |
LAQPKDLYOBZWBT-NYLDSJSYSA-N | |
Source | PubChem | |
URL | https://pubchem.ncbi.nlm.nih.gov | |
Description | Data deposited in or computed by PubChem | |
Canonical SMILES |
CC1C(C(C(C(O1)OC(C(C=O)NC(=O)C)C(C(CO)O)OC2C(C(C(C(O2)CO)O)OC3(CC(C(C(O3)C(C(CO)O)O)NC(=O)C)O)C(=O)O)O)O)O)O | |
Source | PubChem | |
URL | https://pubchem.ncbi.nlm.nih.gov | |
Description | Data deposited in or computed by PubChem | |
Isomeric SMILES |
C[C@H]1[C@H]([C@H]([C@@H]([C@@H](O1)O[C@H]([C@H](C=O)NC(=O)C)[C@@H]([C@@H](CO)O)O[C@H]2[C@@H]([C@H]([C@H]([C@H](O2)CO)O)O[C@@]3(C[C@@H]([C@H]([C@@H](O3)[C@@H]([C@@H](CO)O)O)NC(=O)C)O)C(=O)O)O)O)O)O | |
Source | PubChem | |
URL | https://pubchem.ncbi.nlm.nih.gov | |
Description | Data deposited in or computed by PubChem | |
Molecular Formula |
C31H52N2O23 | |
Source | PubChem | |
URL | https://pubchem.ncbi.nlm.nih.gov | |
Description | Data deposited in or computed by PubChem | |
DSSTOX Substance ID |
DTXSID60913167 | |
Record name | 3′-Sialyl-Lewis X | |
Source | EPA DSSTox | |
URL | https://comptox.epa.gov/dashboard/DTXSID60913167 | |
Description | DSSTox provides a high quality public chemistry resource for supporting improved predictive toxicology. | |
Molecular Weight |
820.7 g/mol | |
Source | PubChem | |
URL | https://pubchem.ncbi.nlm.nih.gov | |
Description | Data deposited in or computed by PubChem | |
Physical Description |
Solid | |
Record name | Sialyl-Lewis X | |
Source | Human Metabolome Database (HMDB) | |
URL | http://www.hmdb.ca/metabolites/HMDB0006565 | |
Description | The Human Metabolome Database (HMDB) is a freely available electronic database containing detailed information about small molecule metabolites found in the human body. | |
Explanation | HMDB is offered to the public as a freely available resource. Use and re-distribution of the data, in whole or in part, for commercial purposes requires explicit permission of the authors and explicit acknowledgment of the source material (HMDB) and the original publication (see the HMDB citing page). We ask that users who download significant portions of the database cite the HMDB paper in any resulting publications. | |
CAS No. |
98603-84-0 | |
Record name | Sialyl-Lewis X | |
Source | CAS Common Chemistry | |
URL | https://commonchemistry.cas.org/detail?cas_rn=98603-84-0 | |
Description | CAS Common Chemistry is an open community resource for accessing chemical information. Nearly 500,000 chemical substances from CAS REGISTRY cover areas of community interest, including common and frequently regulated chemicals, and those relevant to high school and undergraduate chemistry classes. This chemical information, curated by our expert scientists, is provided in alignment with our mission as a division of the American Chemical Society. | |
Explanation | The data from CAS Common Chemistry is provided under a CC-BY-NC 4.0 license, unless otherwise stated. | |
Record name | Sialyl lewis X | |
Source | ChemIDplus | |
URL | https://pubchem.ncbi.nlm.nih.gov/substance/?source=chemidplus&sourceid=0098603840 | |
Description | ChemIDplus is a free, web search system that provides access to the structure and nomenclature authority files used for the identification of chemical substances cited in National Library of Medicine (NLM) databases, including the TOXNET system. | |
Record name | 3′-Sialyl-Lewis X | |
Source | EPA DSSTox | |
URL | https://comptox.epa.gov/dashboard/DTXSID60913167 | |
Description | DSSTox provides a high quality public chemistry resource for supporting improved predictive toxicology. | |
Record name | SIALYL LEWIS X | |
Source | FDA Global Substance Registration System (GSRS) | |
URL | https://gsrs.ncats.nih.gov/ginas/app/beta/substances/0PS35WG8U3 | |
Description | The FDA Global Substance Registration System (GSRS) enables the efficient and accurate exchange of information on what substances are in regulated products. Instead of relying on names, which vary across regulatory domains, countries, and regions, the GSRS knowledge base makes it possible for substances to be defined by standardized, scientific descriptions. | |
Explanation | Unless otherwise noted, the contents of the FDA website (www.fda.gov), both text and graphics, are not copyrighted. They are in the public domain and may be republished, reprinted and otherwise used freely by anyone without the need to obtain permission from FDA. Credit to the U.S. Food and Drug Administration as the source is appreciated but not required. | |
Record name | Sialyl-Lewis X | |
Source | Human Metabolome Database (HMDB) | |
URL | http://www.hmdb.ca/metabolites/HMDB0006565 | |
Description | The Human Metabolome Database (HMDB) is a freely available electronic database containing detailed information about small molecule metabolites found in the human body. | |
Explanation | HMDB is offered to the public as a freely available resource. Use and re-distribution of the data, in whole or in part, for commercial purposes requires explicit permission of the authors and explicit acknowledgment of the source material (HMDB) and the original publication (see the HMDB citing page). We ask that users who download significant portions of the database cite the HMDB paper in any resulting publications. | |
Retrosynthesis Analysis
AI-Powered Synthesis Planning: Our tool employs the Template_relevance Pistachio, Template_relevance Bkms_metabolic, Template_relevance Pistachio_ringbreaker, Template_relevance Reaxys, Template_relevance Reaxys_biocatalysis model, leveraging a vast database of chemical reactions to predict feasible synthetic routes.
One-Step Synthesis Focus: Specifically designed for one-step synthesis, it provides concise and direct routes for your target compounds, streamlining the synthesis process.
Accurate Predictions: Utilizing the extensive PISTACHIO, BKMS_METABOLIC, PISTACHIO_RINGBREAKER, REAXYS, REAXYS_BIOCATALYSIS database, our tool offers high-accuracy predictions, reflecting the latest in chemical research and data.
Strategy Settings
Precursor scoring | Relevance Heuristic |
---|---|
Min. plausibility | 0.01 |
Model | Template_relevance |
Template Set | Pistachio/Bkms_metabolic/Pistachio_ringbreaker/Reaxys/Reaxys_biocatalysis |
Top-N result to add to graph | 6 |
Feasible Synthetic Routes
Disclaimer and Information on In-Vitro Research Products
Please be aware that all articles and product information presented on BenchChem are intended solely for informational purposes. The products available for purchase on BenchChem are specifically designed for in-vitro studies, which are conducted outside of living organisms. In-vitro studies, derived from the Latin term "in glass," involve experiments performed in controlled laboratory settings using cells or tissues. It is important to note that these products are not categorized as medicines or drugs, and they have not received approval from the FDA for the prevention, treatment, or cure of any medical condition, ailment, or disease. We must emphasize that any form of bodily introduction of these products into humans or animals is strictly prohibited by law. It is essential to adhere to these guidelines to ensure compliance with legal and ethical standards in research and experimentation.