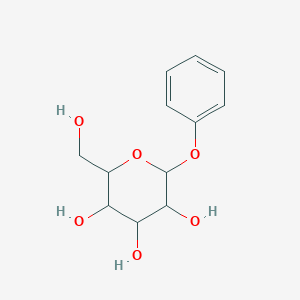
Phenyl alpha-D-glucopyranoside
Overview
Description
Phenyl alpha-D-glucopyranoside is a glycoside compound where a phenyl group is attached to the anomeric carbon of alpha-D-glucopyranose. This compound is known for its role in various biochemical processes and its applications in scientific research. It is a derivative of glucose, a fundamental carbohydrate in energy metabolism.
Mechanism of Action
- Phenyl alpha-D-glucopyranoside (also known as phenyl α-D-glucoside) is a natural compound found in certain plants . Its primary targets are not extensively studied, but it exhibits anti-inflammatory activity.
- In particular, it inhibits nitric oxide (NO) production and suppresses the expression of inducible NO synthase (iNOS) and cyclooxygenase-2 (COX-2) in murine Raw 264.7 macrophages .
- This compound interacts with inflammatory pathways. It attenuates proinflammatory cytokines (such as tumor necrosis factor-α and interleukin-1β) and other inflammation-related genes .
- Additionally, it inhibits increased adhesion, ninjurin 1 (Ninj1) expression, and matrix metalloproteinase (MMP) activity induced by endotoxin treatment .
- The compound’s anti-inflammatory effects are attributed to its interference with nuclear factor-κB (NF-κB) nuclear translocation, a key step in the inflammatory process .
- This compound impacts pathways related to inflammation, cytokine production, and adhesion molecules .
Target of Action
Mode of Action
Biochemical Pathways
Result of Action
Biochemical Analysis
Biochemical Properties
Phenyl alpha-D-glucopyranoside interacts with various enzymes and proteins. For instance, it is hydrolyzed by the enzyme alpha-glucosidase . The nature of these interactions involves the formation of hydrogen bonds between the hydroxyl groups of this compound and the active sites of the enzymes .
Cellular Effects
This compound has been found to exhibit anti-inflammatory activity in lipopolysaccharide-activated RAW 264.7 cells . It inhibits the production of nitric oxide and significantly reduces the expression of inducible nitric oxide synthase (iNOS) and cyclooxygenase-2 (COX-2) . Furthermore, it attenuates proinflammatory cytokines, including tumor necrosis factor-α (TNF-α), interleukin-1β (IL-1β), and other inflammation-related genes, such as IL-6 .
Molecular Mechanism
The molecular mechanism of action of this compound involves its binding interactions with biomolecules and its influence on gene expression. It inhibits the nuclear translocation of nuclear factor-κB (NF-κB), a key transcription factor involved in the inflammatory process . This inhibition results in a decrease in the transcription of numerous target genes, such as pro-inflammatory cytokines and adhesion molecules .
Temporal Effects in Laboratory Settings
It is known that the compound forms dimers with different structures, maintaining the same intramolecular interactions of the monomers but with additional intermolecular interactions between the hydroxyl groups .
Metabolic Pathways
It is known to interact with the enzyme alpha-glucosidase, suggesting its involvement in carbohydrate metabolism .
Preparation Methods
Synthetic Routes and Reaction Conditions: Phenyl alpha-D-glucopyranoside can be synthesized through the reaction of alpha-D-glucopyranose with phenol in the presence of an acid catalyst. The reaction typically involves heating the mixture to promote the formation of the glycosidic bond.
Industrial Production Methods: In industrial settings, the synthesis of this compound may involve more efficient catalytic processes and optimized reaction conditions to increase yield and purity. The use of continuous flow reactors and advanced purification techniques can enhance the scalability of production.
Chemical Reactions Analysis
Types of Reactions: Phenyl alpha-D-glucopyranoside undergoes various chemical reactions, including hydrolysis, oxidation, and substitution.
Common Reagents and Conditions:
Hydrolysis: Acidic or enzymatic conditions can hydrolyze the glycosidic bond, yielding phenol and alpha-D-glucopyranose.
Oxidation: Oxidizing agents such as potassium permanganate can oxidize the phenyl group, leading to the formation of this compound derivatives.
Substitution: Nucleophilic substitution reactions can occur at the phenyl group, introducing different functional groups.
Major Products: The major products formed from these reactions include phenol, alpha-D-glucopyranose, and various substituted this compound derivatives.
Scientific Research Applications
Phenyl alpha-D-glucopyranoside has a wide range of applications in scientific research:
Chemistry: It is used as a model compound to study glycosidic bond formation and cleavage.
Biology: It serves as a substrate in enzymatic assays to investigate the activity of glycosidases.
Industry: It is utilized in the synthesis of other glycoside compounds and as a building block in the production of complex carbohydrates.
Comparison with Similar Compounds
- Phenyl beta-D-glucopyranoside
- Phenyl beta-D-galactopyranoside
- 4-nitrophenyl alpha-D-glucopyranoside
These compounds share structural similarities but differ in their glycosidic bond configuration and functional group substitutions, leading to varied biological activities and applications.
Properties
IUPAC Name |
2-(hydroxymethyl)-6-phenoxyoxane-3,4,5-triol | |
---|---|---|
Details | Computed by LexiChem 2.6.6 (PubChem release 2019.06.18) | |
Source | PubChem | |
URL | https://pubchem.ncbi.nlm.nih.gov | |
Description | Data deposited in or computed by PubChem | |
InChI |
InChI=1S/C12H16O6/c13-6-8-9(14)10(15)11(16)12(18-8)17-7-4-2-1-3-5-7/h1-5,8-16H,6H2 | |
Details | Computed by InChI 1.0.5 (PubChem release 2019.06.18) | |
Source | PubChem | |
URL | https://pubchem.ncbi.nlm.nih.gov | |
Description | Data deposited in or computed by PubChem | |
InChI Key |
NEZJDVYDSZTRFS-UHFFFAOYSA-N | |
Details | Computed by InChI 1.0.5 (PubChem release 2019.06.18) | |
Source | PubChem | |
URL | https://pubchem.ncbi.nlm.nih.gov | |
Description | Data deposited in or computed by PubChem | |
Canonical SMILES |
C1=CC=C(C=C1)OC2C(C(C(C(O2)CO)O)O)O | |
Details | Computed by OEChem 2.1.5 (PubChem release 2019.06.18) | |
Source | PubChem | |
URL | https://pubchem.ncbi.nlm.nih.gov | |
Description | Data deposited in or computed by PubChem | |
Molecular Formula |
C12H16O6 | |
Details | Computed by PubChem 2.1 (PubChem release 2019.06.18) | |
Source | PubChem | |
URL | https://pubchem.ncbi.nlm.nih.gov | |
Description | Data deposited in or computed by PubChem | |
DSSTOX Substance ID |
DTXSID40863030 | |
Record name | Phenyl hexopyranoside | |
Source | EPA DSSTox | |
URL | https://comptox.epa.gov/dashboard/DTXSID40863030 | |
Description | DSSTox provides a high quality public chemistry resource for supporting improved predictive toxicology. | |
Molecular Weight |
256.25 g/mol | |
Details | Computed by PubChem 2.1 (PubChem release 2021.05.07) | |
Source | PubChem | |
URL | https://pubchem.ncbi.nlm.nih.gov | |
Description | Data deposited in or computed by PubChem | |
CAS No. |
4630-62-0 | |
Record name | NSC226967 | |
Source | DTP/NCI | |
URL | https://dtp.cancer.gov/dtpstandard/servlet/dwindex?searchtype=NSC&outputformat=html&searchlist=226967 | |
Description | The NCI Development Therapeutics Program (DTP) provides services and resources to the academic and private-sector research communities worldwide to facilitate the discovery and development of new cancer therapeutic agents. | |
Explanation | Unless otherwise indicated, all text within NCI products is free of copyright and may be reused without our permission. Credit the National Cancer Institute as the source. | |
Retrosynthesis Analysis
AI-Powered Synthesis Planning: Our tool employs the Template_relevance Pistachio, Template_relevance Bkms_metabolic, Template_relevance Pistachio_ringbreaker, Template_relevance Reaxys, Template_relevance Reaxys_biocatalysis model, leveraging a vast database of chemical reactions to predict feasible synthetic routes.
One-Step Synthesis Focus: Specifically designed for one-step synthesis, it provides concise and direct routes for your target compounds, streamlining the synthesis process.
Accurate Predictions: Utilizing the extensive PISTACHIO, BKMS_METABOLIC, PISTACHIO_RINGBREAKER, REAXYS, REAXYS_BIOCATALYSIS database, our tool offers high-accuracy predictions, reflecting the latest in chemical research and data.
Strategy Settings
Precursor scoring | Relevance Heuristic |
---|---|
Min. plausibility | 0.01 |
Model | Template_relevance |
Template Set | Pistachio/Bkms_metabolic/Pistachio_ringbreaker/Reaxys/Reaxys_biocatalysis |
Top-N result to add to graph | 6 |
Feasible Synthetic Routes
Disclaimer and Information on In-Vitro Research Products
Please be aware that all articles and product information presented on BenchChem are intended solely for informational purposes. The products available for purchase on BenchChem are specifically designed for in-vitro studies, which are conducted outside of living organisms. In-vitro studies, derived from the Latin term "in glass," involve experiments performed in controlled laboratory settings using cells or tissues. It is important to note that these products are not categorized as medicines or drugs, and they have not received approval from the FDA for the prevention, treatment, or cure of any medical condition, ailment, or disease. We must emphasize that any form of bodily introduction of these products into humans or animals is strictly prohibited by law. It is essential to adhere to these guidelines to ensure compliance with legal and ethical standards in research and experimentation.