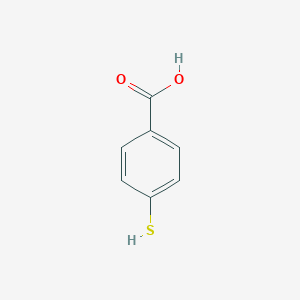
4-Mercaptobenzoic acid
Overview
Description
4-Mercaptobenzoic acid (4-MBA) is a bifunctional molecule containing a thiol (-SH) group and a carboxylic acid (-COOH) group. This structure enables it to form self-assembled monolayers (SAMs) on gold and silver surfaces, making it a cornerstone in surface-enhanced Raman spectroscopy (SERS) applications . The thiol group provides strong chemisorption to metallic nanoparticles, while the carboxylic acid group imparts pH sensitivity, allowing 4-MBA to act as a reporter for intracellular pH measurements . Its large Raman cross-section and resistance to photobleaching further enhance its utility in biological imaging .
Preparation Methods
Conventional Preparation Methods
Esterification of 4-Thiosalicylic Acid
The earliest reported method involves sulfuric acid-catalyzed esterification of 4-thiosalicylic acid with methanol under reflux. This 13-hour reaction yields 4-mercaptobenzoic acid methyl ester, which requires subsequent hydrolysis to isolate the free acid . While straightforward, this approach suffers from prolonged reaction times and side reactions due to the sensitivity of thiol groups under acidic conditions.
Grignard Reaction with 4-Bromothiophenol
A multistep synthesis employs 4-bromothiophenol, which undergoes a Grignard reaction with methylmagnesium chloride to form 4-mercaptophenylmagnesium bromide. Quenching with methyl chloroformate produces the methyl ester, followed by acid hydrolysis . This method demands anhydrous conditions, cryogenic temperatures, and careful handling of organometallic reagents, rendering it impractical for large-scale applications.
Cyano Group Displacement with Potassium Sulfide
4-Cyanobenzene reacts with potassium sulfide in dimethylformamide (DMF), yielding 4,4'-dicyanodiphenyl sulfide and 4-cyanothiophenol. Subsequent esterification with methanol and sulfuric acid generates this compound derivatives . However, competing side reactions and the need for toxic sulfide reagents limit its utility.
Novel Industrial Synthesis via Halogenation-Esterification
Substitution Reaction of 4-Chlorobenzoic Acid
The patented industrial method begins with 4-chlorobenzoic acid, which reacts with sodium methyl mercaptide in DMF at 70°C for 5 hours (Equation 1):
Acidification with HCl precipitates 4-methylthiobenzoic acid in 88% yield. This step avoids thiol oxidation by deferring deprotection until later stages.
Halogenation of Methylthiobenzoic Acid
The methylthio intermediate undergoes chlorination in ethylene dichloride at 10–20°C, yielding 4-trichloromethylthiobenzoic acid (Equation 2):
Controlling chlorine gas flow and temperature minimizes overhalogenation, achieving 87.5% isolated yield. Ethylene dichloride is preferred for its low polarity, which reduces side reactions.
Alcoholysis and Hydrolysis
Trichloromethylthiobenzoic acid reacts with methanol at 60°C, undergoing nucleophilic displacement to form methyl 4-mercaptobenzoate (Equation 3):
Base-catalyzed hydrolysis of the ester liberates 4-MBA in 90% yield. This one-pot sequence eliminates purification of intermediates, enhancing process efficiency.
Comparative Analysis of Synthesis Methods
Reaction Efficiency and Scalability
The industrial halogenation-esterification route outperforms conventional methods in yield (85–90% vs. 50–70%) and reaction time (12 hours total vs. 24–48 hours). By avoiding Grignard reagents and cryogenic conditions, it reduces operational complexity and energy consumption .
Data Tables: Synthesis Parameters and Outcomes
Table 1. Comparison of Key Reaction Conditions
Method | Temperature (°C) | Time (h) | Yield (%) | Key Reagents |
---|---|---|---|---|
Esterification | 70 | 13 | 65 | H₂SO₄, MeOH |
Grignard | -30 | 8 | 58 | Mg, MeMgCl |
Industrial Patent | 60 | 6 | 90 | NaSCH₃, Cl₂, MeOH |
Table 2. Byproduct Generation Across Methods
Chemical Reactions Analysis
Types of Reactions: 4-Mercaptobenzoic acid undergoes various chemical reactions, including oxidation, reduction, and substitution reactions .
Common Reagents and Conditions:
Major Products:
Oxidation: Disulfides (e.g., 4,4’-dithiobisbenzoic acid).
Reduction: Thiol derivatives.
Substitution: Various substituted benzoic acids.
Scientific Research Applications
Surface-Enhanced Raman Scattering (SERS)
Overview:
4-MBA is widely used as a SERS probe due to its strong affinity for metal surfaces, particularly gold and silver. The compound enhances the Raman signal of analytes, making it useful for detecting low concentrations of substances.
Case Study:
A study investigated the use of 4-MBA labeled gold-silver-alloy-embedded silica nanoparticles for the detection of thiram, a pesticide. The SERS intensity was optimized by varying the concentration of 4-MBA and thiram, achieving high sensitivity at concentrations as low as 1 µM. The results demonstrated that the SERS signal was significantly enhanced when using 4-MBA as an internal standard, highlighting its effectiveness in pesticide detection .
Photovoltaic Applications
Overview:
In photovoltaic technology, 4-MBA serves as a bifunctional linker molecule that enhances the performance of quantum dot (QD) solar cells. It facilitates the attachment of QDs to TiO2 films, improving charge transfer rates.
Case Study:
Research focused on a novel bifunctional linker molecule involving 4-MBA showed improved photovoltaic performance in PbS QD solar cells. The study indicated that using 4-MBA as a linker resulted in higher open-circuit voltage (V_oc) and power conversion efficiency compared to traditional linkers . This advancement suggests that 4-MBA can significantly enhance the efficiency of solar energy conversion technologies.
Drug Delivery Systems
Overview:
4-MBA has been explored in drug delivery systems due to its chemical properties that allow for functionalization and controlled release mechanisms.
Case Study:
A study developed a floating drug delivery system using hydrophobic coated buoyant cores incorporating 4-MBA. This system demonstrated sustained release characteristics for drugs like cisapride, showcasing the potential of 4-MBA in enhancing drug delivery efficacy through innovative formulation strategies .
Environmental Monitoring
Overview:
The compound is also utilized in environmental monitoring applications, particularly for detecting food contaminants and hazardous substances.
Case Study:
Research highlighted the use of 4-MBA in developing advanced analytical methods for sensing food contaminants. The study demonstrated that SERS substrates functionalized with 4-MBA could effectively detect various foodborne pathogens and contaminants at low concentrations, underscoring its role in food safety monitoring .
Nanotechnology Applications
Overview:
In nanotechnology, 4-MBA is employed to create self-assembled monolayers (SAMs) on metal surfaces, which are crucial for various nanostructured devices.
Case Study:
A theoretical study examined the assembly of 4-MBA on silver surfaces for SERS applications. The findings indicated that SAMs formed with 4-MBA could significantly reduce trap states and enhance charge transfer rates in quantum dot ensembles, making them suitable for applications in nanodevices .
Data Summary Table
Application Area | Description | Key Findings |
---|---|---|
Surface-Enhanced Raman Scattering | Used as a probe for detecting pesticides like thiram | High sensitivity achieved at low concentrations (1 µM) |
Photovoltaic Technology | Acts as a linker molecule in QD solar cells | Improved V_oc and efficiency compared to traditional linkers |
Drug Delivery Systems | Incorporated into floating drug delivery systems | Sustained release characteristics demonstrated |
Environmental Monitoring | Utilized for detecting food contaminants | Effective detection at low concentrations |
Nanotechnology | Forms self-assembled monolayers on metal surfaces | Enhances charge transfer rates and reduces trap states |
Mechanism of Action
The mechanism of action of 4-mercaptobenzoic acid involves its ability to form strong bonds with metal surfaces through the thiol group. This property is exploited in SERS, where the compound enhances the Raman signal of analytes by forming a self-assembled monolayer on metal nanoparticles . The carboxylic acid group can also participate in hydrogen bonding and electrostatic interactions, contributing to its versatility in various applications .
Comparison with Similar Compounds
Comparison with Structurally Similar Compounds
4-Hydroxybenzoic Acid
- Structure : Contains a hydroxyl (-OH) group instead of a thiol (-SH) group.
- Key Differences: Lacks the thiol group, preventing effective SAM formation on metal surfaces. Less versatile in redox reactions compared to 4-MBA due to the absence of the -SH group .
4-(2-Hydroxyethyl)benzoic Acid
- Structure : Features a hydroxyethyl (-CH2CH2OH) group instead of -SH.
- Key Differences :
- The hydroxyethyl group reduces chemical reactivity and metal-binding capacity.
- Shows weaker enzyme interactions compared to 4-MBA, limiting its use in biosensing .
Thiophenol (TP) and 4-Mercaptophenol (MP)
- Structure : TP has a benzene ring with -SH; MP adds a -OH group.
- Key Differences :
4-Mercaptophenylboronic Acid (MPBA)
- Structure : Contains a boronic acid (-B(OH)2) group instead of -COOH.
- Key Differences :
4-Nitrobenzenethiol
- Structure: Features a nitro (-NO2) group instead of -COOH.
- Key Differences :
pH Sensitivity
The -COOH group in 4-MBA undergoes protonation/deprotonation (COOH ↔ COO⁻) in response to pH changes, altering its SERS spectrum. Key spectral markers include:
- ν(COO⁻) stretch at 1380 cm⁻¹ and 1410 cm⁻¹ (deprotonated state).
- ν(COOH) stretch at ~1700 cm⁻¹ (protonated state).
- ν8a ring breathing mode at ~1580 cm⁻¹, which shifts systematically with pH .
Comparison : Compounds like TP or MP lack these pH-sensitive features, making 4-MBA uniquely suited for intracellular pH mapping .
Metal Ion Detection
4-MBA's -COOH group enhances coordination with metal ions (e.g., Cr³⁺), enabling colorimetric detection with a limit of detection (LOD) of 5 × 10⁻⁶ M. In contrast, 4-nitrobenzenethiol-based probes show lower sensitivity due to the absence of -COOH .
SERS Performance
- Enhancement Factor: 4-MBA-functionalized gold nanostars achieve a Raman enhancement factor of ~10⁷, outperforming spherical nanoparticles .
- Stability : 4-MBA SAMs resist decarboxylation under physiological conditions, unlike MPBA, which degrades in basic environments .
Research Findings and Methodological Insights
- Intracellular pH Mapping: 4-MBA-functionalized gold nanostars enable precise pH determination via ν8a frequency shifts, with minimal interference from cellular autofluorescence .
- Decarboxylation Resistance : 4-MBA retains its -COOH group under physiological conditions, unlike analogs prone to decarboxylation (e.g., MPBA) .
- Multi-Modal Sensing : The combination of ν(COO⁻) intensity ratios and ν8a shifts allows cross-verification of pH measurements, enhancing reliability .
Biological Activity
4-Mercaptobenzoic acid (4-MBA) is a compound that has garnered significant interest in various fields of research, particularly for its biological activities and applications in nanotechnology and sensing. This article provides a comprehensive overview of the biological activity of 4-MBA, including its mechanisms, applications, and findings from recent studies.
4-MBA is characterized by its thiol (-SH) group attached to a benzoic acid structure. Its chemical formula is C₇H₆O₂S, and it has a molecular weight of 154.18 g/mol. The presence of the thiol group allows for unique interactions with metal nanoparticles, making it a valuable compound in surface-enhanced Raman spectroscopy (SERS) and other applications.
The biological activity of 4-MBA can be attributed to several mechanisms:
- SERS Applications : 4-MBA is extensively used as a pH-sensitive reporter molecule in SERS applications. It allows for the detection of intracellular pH changes, which can indicate various cellular processes, including cancer progression and drug delivery efficacy . The SERS spectra of 4-MBA reveal peaks corresponding to different vibrational modes that shift with pH changes, enabling precise measurements.
- Nanoparticle Functionalization : 4-MBA is often used to functionalize gold and silver nanoparticles, enhancing their stability and biocompatibility. This functionalization facilitates targeted drug delivery systems and biosensing platforms .
- Cellular Interactions : Studies have shown that 4-MBA interacts with cellular membranes and can influence cellular uptake mechanisms. For instance, when conjugated with cell-penetrating peptides, 4-MBA-functionalized nanoparticles demonstrated improved internalization in cancer cells .
Biological Effects
Research has highlighted several biological effects associated with 4-MBA:
- Antioxidant Properties : Some studies suggest that 4-MBA exhibits antioxidant activity, which can protect cells from oxidative stress. This property may have implications for its use in therapeutic applications aimed at reducing cellular damage .
- Cytotoxicity : While 4-MBA is generally considered biocompatible, its cytotoxic effects can vary based on concentration and exposure duration. Research indicates that at higher concentrations, it may induce apoptosis in certain cell lines .
Case Studies and Research Findings
- Intracellular pH Sensing : A study utilized 4-MBA-functionalized gold nanostars for intracellular pH sensing in SW620 cancer cells. The results indicated that the SERS signals could accurately reflect pH changes within the cells, demonstrating the potential of 4-MBA in monitoring cellular environments .
- Nanoparticle Stability : In another investigation, the stability of 4-MBA-coated nanoparticles was assessed under various conditions. The findings showed that the coating significantly improved the nanoparticles' resistance to aggregation and degradation, enhancing their effectiveness as drug delivery vehicles .
- Photovoltaic Applications : Research exploring the use of 4-MBA as a bifunctional linker in quantum dot solar cells revealed that it could enhance binding strength and efficiency compared to traditional linkers. This application underscores the versatility of 4-MBA beyond biological contexts .
Data Table: Summary of Biological Activities
Q & A
Basic Research Questions
Q. How is 4-MBA synthesized and characterized for use in surface-enhanced Raman spectroscopy (SERS)?
- Methodology : 4-MBA is typically synthesized via thiolation of 4-bromobenzoic acid using thiourea, followed by hydrolysis. Characterization involves nuclear magnetic resonance (NMR) for purity verification and Fourier-transform infrared spectroscopy (FTIR) to confirm the thiol (-SH) and carboxylic (-COOH) functional groups . For SERS applications, 4-MBA is self-assembled onto gold or silver nanoparticles (Au/AgNPs) through thiolate-metal bonding. Transmission electron microscopy (TEM) and UV-Vis spectroscopy are used to confirm nanoparticle functionalization .
- Key Data :
- Molecular formula: C₇H₆O₂S .
- Characteristic Raman peaks: ~1,080 cm⁻¹ (C-S stretch) and ~1,580 cm⁻¹ (aromatic ring vibrations) .
Q. What experimental protocols ensure reproducibility in preparing 4-MBA self-assembled monolayers (SAMs)?
- Methodology : SAMs are formed by immersing Au/Ag substrates in 1 mM 4-MBA ethanolic solutions for 12–24 hours. Excess molecules are rinsed with ethanol. Electrochemical impedance spectroscopy (EIS) or cyclic voltammetry (CV) validates monolayer integrity by measuring electron transfer resistance .
- Critical Factors :
- pH adjustment (e.g., pH 4 for hydrogen bonding-driven nanoparticle assembly) enhances SAM stability .
- Temperature control (25°C) minimizes aggregation during functionalization .
Advanced Research Questions
Q. How do 4-MBA-stabilized gold nanoparticles exhibit enhanced electrochemical kinetics compared to citrate-stabilized counterparts?
- Methodology : Cyclic voltammetry (CV) and chronoamperometry compare electron transfer rates. For example, 4-MBA-functionalized AuNPs show a 71 mV lower oxidation potential and twice the reaction speed of citrate-stabilized AuNPs due to stronger ligand-metal interactions .
- Data Contradiction Analysis : Discrepancies in reaction rates may arise from variations in SAM packing density. Atomic force microscopy (AFM) or X-ray photoelectron spectroscopy (XPS) can resolve structural differences .
Q. What mechanisms explain the pH-dependent SERS enhancement of 4-MBA in nanoparticle "hot spots"?
- Methodology : Adjusting pH to 4 induces hydrogen bonding between carboxylic groups of 4-MBA on adjacent nanoparticles, creating sub-1 nm gaps for plasmonic enhancement. Single-molecule SERS experiments (e.g., using a 785 nm laser) detect signal amplification up to 10¹⁴–10¹⁵ times .
- Theoretical Modeling : Density functional theory (DFT) simulations correlate protonation states of 4-MBA with Raman peak shifts. For instance, deprotonated -COO⁻ groups at pH > 5 reduce hydrogen bonding, diminishing hot-spot formation .
Q. How can computational models predict the orientation of 4-MBA molecules in SAMs for biosensing applications?
- Methodology : Molecular dynamics (MD) simulations using software like GROMACS model SAMs on Au(111) surfaces. Parameters include tilt angles (e.g., 30–40° from surface normal) and sulfur-gold bond distances (~2.4 Å). Experimental validation uses polarization-modulated infrared reflection absorption spectroscopy (PM-IRRAS) .
- Key Findings :
- Carboxylic groups orient toward the solution, enabling covalent immobilization of biomolecules (e.g., glucose oxidase) .
Q. Data Contradiction and Resolution
Q. Why do studies report conflicting SERS intensities for 4-MBA under similar experimental conditions?
- Analysis : Variations arise from nanoparticle size/shape heterogeneity (e.g., spherical vs. nanostar AuNPs) and laser wavelength alignment with plasmon resonance. Standardizing substrates (e.g., using template-synthesized Ag nanowires) reduces variability .
- Resolution Strategy : Normalize SERS signals using internal reference peaks (e.g., 4-MBA’s 1,080 cm⁻¹ band) and report enhancement factors (EFs) with error margins .
Properties
IUPAC Name |
4-sulfanylbenzoic acid | |
---|---|---|
Source | PubChem | |
URL | https://pubchem.ncbi.nlm.nih.gov | |
Description | Data deposited in or computed by PubChem | |
InChI |
InChI=1S/C7H6O2S/c8-7(9)5-1-3-6(10)4-2-5/h1-4,10H,(H,8,9) | |
Source | PubChem | |
URL | https://pubchem.ncbi.nlm.nih.gov | |
Description | Data deposited in or computed by PubChem | |
InChI Key |
LMJXSOYPAOSIPZ-UHFFFAOYSA-N | |
Source | PubChem | |
URL | https://pubchem.ncbi.nlm.nih.gov | |
Description | Data deposited in or computed by PubChem | |
Canonical SMILES |
C1=CC(=CC=C1C(=O)O)S | |
Source | PubChem | |
URL | https://pubchem.ncbi.nlm.nih.gov | |
Description | Data deposited in or computed by PubChem | |
Molecular Formula |
C7H6O2S | |
Source | PubChem | |
URL | https://pubchem.ncbi.nlm.nih.gov | |
Description | Data deposited in or computed by PubChem | |
DSSTOX Substance ID |
DTXSID40148036 | |
Record name | 4-Mercaptobenzoate | |
Source | EPA DSSTox | |
URL | https://comptox.epa.gov/dashboard/DTXSID40148036 | |
Description | DSSTox provides a high quality public chemistry resource for supporting improved predictive toxicology. | |
Molecular Weight |
154.19 g/mol | |
Source | PubChem | |
URL | https://pubchem.ncbi.nlm.nih.gov | |
Description | Data deposited in or computed by PubChem | |
CAS No. |
1074-36-8 | |
Record name | 4-Mercaptobenzoic acid | |
Source | CAS Common Chemistry | |
URL | https://commonchemistry.cas.org/detail?cas_rn=1074-36-8 | |
Description | CAS Common Chemistry is an open community resource for accessing chemical information. Nearly 500,000 chemical substances from CAS REGISTRY cover areas of community interest, including common and frequently regulated chemicals, and those relevant to high school and undergraduate chemistry classes. This chemical information, curated by our expert scientists, is provided in alignment with our mission as a division of the American Chemical Society. | |
Explanation | The data from CAS Common Chemistry is provided under a CC-BY-NC 4.0 license, unless otherwise stated. | |
Record name | 4-Mercaptobenzoate | |
Source | ChemIDplus | |
URL | https://pubchem.ncbi.nlm.nih.gov/substance/?source=chemidplus&sourceid=0001074368 | |
Description | ChemIDplus is a free, web search system that provides access to the structure and nomenclature authority files used for the identification of chemical substances cited in National Library of Medicine (NLM) databases, including the TOXNET system. | |
Record name | 1074-36-8 | |
Source | DTP/NCI | |
URL | https://dtp.cancer.gov/dtpstandard/servlet/dwindex?searchtype=NSC&outputformat=html&searchlist=32022 | |
Description | The NCI Development Therapeutics Program (DTP) provides services and resources to the academic and private-sector research communities worldwide to facilitate the discovery and development of new cancer therapeutic agents. | |
Explanation | Unless otherwise indicated, all text within NCI products is free of copyright and may be reused without our permission. Credit the National Cancer Institute as the source. | |
Record name | 4-Mercaptobenzoate | |
Source | EPA DSSTox | |
URL | https://comptox.epa.gov/dashboard/DTXSID40148036 | |
Description | DSSTox provides a high quality public chemistry resource for supporting improved predictive toxicology. | |
Retrosynthesis Analysis
AI-Powered Synthesis Planning: Our tool employs the Template_relevance Pistachio, Template_relevance Bkms_metabolic, Template_relevance Pistachio_ringbreaker, Template_relevance Reaxys, Template_relevance Reaxys_biocatalysis model, leveraging a vast database of chemical reactions to predict feasible synthetic routes.
One-Step Synthesis Focus: Specifically designed for one-step synthesis, it provides concise and direct routes for your target compounds, streamlining the synthesis process.
Accurate Predictions: Utilizing the extensive PISTACHIO, BKMS_METABOLIC, PISTACHIO_RINGBREAKER, REAXYS, REAXYS_BIOCATALYSIS database, our tool offers high-accuracy predictions, reflecting the latest in chemical research and data.
Strategy Settings
Precursor scoring | Relevance Heuristic |
---|---|
Min. plausibility | 0.01 |
Model | Template_relevance |
Template Set | Pistachio/Bkms_metabolic/Pistachio_ringbreaker/Reaxys/Reaxys_biocatalysis |
Top-N result to add to graph | 6 |
Feasible Synthetic Routes
Disclaimer and Information on In-Vitro Research Products
Please be aware that all articles and product information presented on BenchChem are intended solely for informational purposes. The products available for purchase on BenchChem are specifically designed for in-vitro studies, which are conducted outside of living organisms. In-vitro studies, derived from the Latin term "in glass," involve experiments performed in controlled laboratory settings using cells or tissues. It is important to note that these products are not categorized as medicines or drugs, and they have not received approval from the FDA for the prevention, treatment, or cure of any medical condition, ailment, or disease. We must emphasize that any form of bodily introduction of these products into humans or animals is strictly prohibited by law. It is essential to adhere to these guidelines to ensure compliance with legal and ethical standards in research and experimentation.