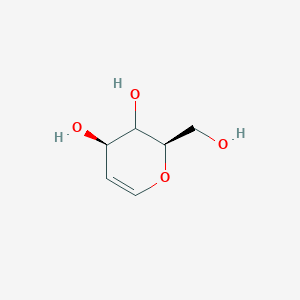
d-Glucal
Overview
Description
d-Glucal is a compound with the molecular formula C6H10O4 . It is also known as 1,5-anhydro-2-deoxy-D-arabino-hex-1-enitol . The structure of d-Glucal consists of a cyclic ketene acetal derived from glucose . This compound is intriguing due to its unique arrangement of functional groups.
Synthesis Analysis
The synthesis of d-Glucal involves the formation of the cyclic ketene acetal ring. Researchers have explored various methods to prepare this compound, including radical ring-opening polymerization of cyclic ketene acetals derived from d-Glucal . Further studies are needed to optimize and expand synthetic routes.
Molecular Structure Analysis
The molecular structure of d-Glucal features a six-membered ring with an oxygen atom bridging two carbon atoms. The hydroxymethyl group and the hydroxyl groups contribute to its distinctive configuration. The IUPAC name for d-Glucal is (2R,3S,4R)-2-(hydroxymethyl)-3,4-dihydro-2H-pyran-3,4-diol .
Physical And Chemical Properties Analysis
Scientific Research Applications
Synthesis of Non-Natural Oligo- and Polysaccharides
d-Glucal is used in α-Glucan Phosphorylase-Catalyzed Enzymatic Reactions to synthesize non-natural oligo- and polysaccharides . By means of weak specificity for the recognition of substrates by α-Glucan Phosphorylase, non-natural oligo- and polysaccharides have precisely been synthesized .
Production of Glycogen-Based Non-Natural Polysaccharide Materials
Glycogen, a highly branched natural polysaccharide, has been used as the polymeric glycosyl acceptor and primer for the α-Glucan Phosphorylase-catalyzed glycosylation and polymerization to obtain glycogen-based non-natural polysaccharide materials .
Synthesis of Amylose Analog Polysaccharides
Under the conditions of removal of inorganic phosphate, thermostable α-Glucan Phosphorylase-catalyzed enzymatic polymerization of analog monomers occurred to give amylose analog polysaccharides .
Building Block for Oligosaccharides Synthesis
d-Glucal is an important building block for both solution- and solid-phase synthesis of oligosaccharides .
Production of 2-Deoxy-α-d-Glucosylated Penta-Heptasaccharides
In the enzymatic reaction in the presence of d-glucal, consecutive glycosylations progressed to produce 2-deoxy-α-d-glucosylated penta-heptasaccharides .
Synthesis of Sporiolide B
d-Glucal has been used in the total synthesis of the 12-membered ring natural macrolide, sporiolide B .
properties
IUPAC Name |
(2R,3S,4R)-2-(hydroxymethyl)-3,4-dihydro-2H-pyran-3,4-diol | |
---|---|---|
Source | PubChem | |
URL | https://pubchem.ncbi.nlm.nih.gov | |
Description | Data deposited in or computed by PubChem | |
InChI |
InChI=1S/C6H10O4/c7-3-5-6(9)4(8)1-2-10-5/h1-2,4-9H,3H2/t4-,5-,6+/m1/s1 | |
Source | PubChem | |
URL | https://pubchem.ncbi.nlm.nih.gov | |
Description | Data deposited in or computed by PubChem | |
InChI Key |
YVECGMZCTULTIS-PBXRRBTRSA-N | |
Source | PubChem | |
URL | https://pubchem.ncbi.nlm.nih.gov | |
Description | Data deposited in or computed by PubChem | |
Canonical SMILES |
C1=COC(C(C1O)O)CO | |
Source | PubChem | |
URL | https://pubchem.ncbi.nlm.nih.gov | |
Description | Data deposited in or computed by PubChem | |
Isomeric SMILES |
C1=CO[C@@H]([C@H]([C@@H]1O)O)CO | |
Source | PubChem | |
URL | https://pubchem.ncbi.nlm.nih.gov | |
Description | Data deposited in or computed by PubChem | |
Molecular Formula |
C6H10O4 | |
Record name | glucal | |
Source | Wikipedia | |
URL | https://en.wikipedia.org/wiki/Glucal | |
Description | Chemical information link to Wikipedia. | |
Source | PubChem | |
URL | https://pubchem.ncbi.nlm.nih.gov | |
Description | Data deposited in or computed by PubChem | |
DSSTOX Substance ID |
DTXSID60157685 | |
Record name | D-(+)-Glucal | |
Source | EPA DSSTox | |
URL | https://comptox.epa.gov/dashboard/DTXSID60157685 | |
Description | DSSTox provides a high quality public chemistry resource for supporting improved predictive toxicology. | |
Molecular Weight |
146.14 g/mol | |
Source | PubChem | |
URL | https://pubchem.ncbi.nlm.nih.gov | |
Description | Data deposited in or computed by PubChem | |
Product Name |
d-Glucal | |
CAS RN |
13265-84-4 | |
Record name | D-(+)-Glucal | |
Source | CAS Common Chemistry | |
URL | https://commonchemistry.cas.org/detail?cas_rn=13265-84-4 | |
Description | CAS Common Chemistry is an open community resource for accessing chemical information. Nearly 500,000 chemical substances from CAS REGISTRY cover areas of community interest, including common and frequently regulated chemicals, and those relevant to high school and undergraduate chemistry classes. This chemical information, curated by our expert scientists, is provided in alignment with our mission as a division of the American Chemical Society. | |
Explanation | The data from CAS Common Chemistry is provided under a CC-BY-NC 4.0 license, unless otherwise stated. | |
Record name | D-(+)-Glucal | |
Source | EPA DSSTox | |
URL | https://comptox.epa.gov/dashboard/DTXSID60157685 | |
Description | DSSTox provides a high quality public chemistry resource for supporting improved predictive toxicology. | |
Record name | 1,5-anhydro-2-deoxy-D-arabino-hex-1-enitol | |
Source | European Chemicals Agency (ECHA) | |
URL | https://echa.europa.eu/substance-information/-/substanceinfo/100.032.949 | |
Description | The European Chemicals Agency (ECHA) is an agency of the European Union which is the driving force among regulatory authorities in implementing the EU's groundbreaking chemicals legislation for the benefit of human health and the environment as well as for innovation and competitiveness. | |
Explanation | Use of the information, documents and data from the ECHA website is subject to the terms and conditions of this Legal Notice, and subject to other binding limitations provided for under applicable law, the information, documents and data made available on the ECHA website may be reproduced, distributed and/or used, totally or in part, for non-commercial purposes provided that ECHA is acknowledged as the source: "Source: European Chemicals Agency, http://echa.europa.eu/". Such acknowledgement must be included in each copy of the material. ECHA permits and encourages organisations and individuals to create links to the ECHA website under the following cumulative conditions: Links can only be made to webpages that provide a link to the Legal Notice page. | |
Retrosynthesis Analysis
AI-Powered Synthesis Planning: Our tool employs the Template_relevance Pistachio, Template_relevance Bkms_metabolic, Template_relevance Pistachio_ringbreaker, Template_relevance Reaxys, Template_relevance Reaxys_biocatalysis model, leveraging a vast database of chemical reactions to predict feasible synthetic routes.
One-Step Synthesis Focus: Specifically designed for one-step synthesis, it provides concise and direct routes for your target compounds, streamlining the synthesis process.
Accurate Predictions: Utilizing the extensive PISTACHIO, BKMS_METABOLIC, PISTACHIO_RINGBREAKER, REAXYS, REAXYS_BIOCATALYSIS database, our tool offers high-accuracy predictions, reflecting the latest in chemical research and data.
Strategy Settings
Precursor scoring | Relevance Heuristic |
---|---|
Min. plausibility | 0.01 |
Model | Template_relevance |
Template Set | Pistachio/Bkms_metabolic/Pistachio_ringbreaker/Reaxys/Reaxys_biocatalysis |
Top-N result to add to graph | 6 |
Feasible Synthetic Routes
Q & A
Q1: How is D-glucal structurally characterized using spectroscopic techniques?
A2: D-Glucal's structure is elucidated using various spectroscopic techniques, including NMR and MS. For instance, [1H NMR spectral data is often employed to confirm the structures of derivatives of functionalized C-β-D-glucopyranosides synthesized from D-glucal. []] Researchers studying the regiochemistry of D-glucal glucosylation utilized FAB MS and MALDI MS to confirm the monoglucosylated nature of the product. [] Additionally, CI MS comparisons with potential regioisomers helped definitively identify the reaction product as D-laminarabial. []
Q2: Does the choice of protecting groups affect the reactivity of D-glucal in Perlin reactions?
A3: Yes, research indicates that the choice of protecting groups significantly influences the outcome of Perlin reactions involving D-glucal. [] Specifically, benzyl and dimethoxyphenylmethyl protecting groups demonstrated compatibility with the reaction conditions, while several silyl protecting groups proved unsuitable. [] This highlights the importance of carefully selecting protecting groups to achieve desired reactivity in D-glucal transformations.
Q3: Can D-glucal act as a glycosyl donor in enzyme-catalyzed reactions?
A4: Yes, D-glucal can function as a glycosyl donor in the presence of certain enzymes. For instance, homogeneous endo-β-1,3-glucanase GA from Cellulomonas cellulans catalyzes the glycosylation of D-glucal using laminarin as the glycosyl residue donor. []
Q4: How do α-glucosidases and exo-α-glucanases differ in their stereochemical outcome when hydrating D-glucal?
A5: Studies revealed distinct stereochemical outcomes in D-glucal hydration depending on the enzyme employed. α-Glucosidases, sourced from various organisms, were found to protonate D-glucal from above its re face, resulting in 2-deoxy-D-glucose with α-configuration. [] In contrast, exo-α-glucanases (glucodextranase and glucoamylase) protonated D-glucal from below its si face. [] This difference highlights the varying mechanisms and active site geometries of these enzymes.
Q5: Can D-glucal participate in reactions catalyzed by alpha-glucan phosphorylases?
A6: Yes, D-glucal can substitute for glucose 1-phosphate as the glucosyl donor in reactions catalyzed by alpha-glucan phosphorylases from various sources. [] Notably, this reaction requires orthophosphate or arsenate and the enzyme must be in its active conformation with pyridoxal 5'-phosphate in its dianionic form. [] The reaction proceeds through a proposed mechanism involving protonation at C-2 of D-glucal, ultimately yielding 2-deoxy-alpha-D-glucose oligo- or polysaccharides. []
Q6: Can D-glucal be used to synthesize C-aryl glucals, and what are the advantages of different palladium-catalyzed methods?
A7: Yes, C-aryl glucals can be synthesized through palladium-catalyzed cross-coupling reactions using D-glucal derivatives. One approach involves coupling 1-tributylstannyl-3,4,6-tri-O-(tert-butyldimethylsilyl)-D-glucal with aryl bromides. [] Alternatively, 1-iodo-3,4,6-tri-O-(triisopropylsilyl)-D-glucal can be reacted with various metalated aromatics like ArZnCl, ArB(OH)2, and ArB(OMe)2. [] This latter method offers advantages such as higher yields, milder conditions, and a more efficient route to the iodo-glucal starting material. []
Q7: How does the structure of the fluorinating agent influence the stereochemical outcome in reactions with D-glucal?
A8: Research indicates that the choice of fluorinating agent significantly affects the stereoselectivity of D-glucal fluorination. For instance, reacting D-glucal with acetyl hypofluorite (AcOF) in a freon solvent (CFCl3) yields predominantly 2-deoxy-2-fluoro-D-glucose (2-FDG), achieving approximately 95% radiochemical purity. [] Conversely, using fluorine gas (F2) or AcOF in acetonitrile leads to the preferential formation of 2-deoxy-2-fluoro-D-mannose (2-FDM) over 2-FDG. [] These observations underscore the importance of carefully selecting the fluorinating agent and solvent to control the stereochemical outcome in D-glucal fluorination reactions.
Q8: Can D-glucal be utilized in the synthesis of 2-deoxy-α-d-glucopyranonucleosides?
A9: Yes, D-glucal serves as a valuable starting material for synthesizing 2-deoxy-α-d-glucopyranonucleosides. [] A key step in this process involves the halogen-mediated O-glycosidation of D-glucal using bromine in methanol. [] Subsequent steps include reductive removal of the halo group and hydrolysis of the methoxy group, ultimately yielding the desired 2-deoxy-d-glucose derivative. [] This approach highlights the versatility of D-glucal in accessing important nucleoside analogues.
Q9: How does the substitution at the C-3 position of D-glucal influence the stereoselectivity of chlorination reactions?
A10: The presence and nature of the substituent at the C-3 position of D-glucal significantly influence the stereochemical outcome of chlorination reactions. [] For example, chlorination of 3-O-acetyl-4,6-O-benzylidene-D-glucal favors β-side attack, leading to the predominant formation of the β-D-manno adduct. [] Conversely, chlorination of 3-deoxy-4,6-O-benzylidene-D-glucal under identical conditions primarily yields the β-D-arabino adduct. [] These findings underscore the importance of considering substituent effects on the stereoselectivity of reactions involving D-glucal derivatives.
Q10: Does the size of the macrocycle affect the biological activity of migrastatin analogues derived from D-glucal?
A11: Yes, the size of the macrocycle significantly influences the biological activity of migrastatin analogues synthesized from D-glucal. [] Studies comparing macrolactone, macrolactam, and macroketone analogues, all derived from D-glucal, revealed that a dorrigocin A analogue exhibited the most potent inhibitory activity against gastric cancer cell migration. [] This finding highlights the importance of exploring structural variations in the macrocycle for optimizing the biological activity of migrastatin analogues.
Q11: Can modifications to the olefin moiety in D-glucal derivatives influence the regioselectivity of hydrostannation reactions?
A12: Yes, the regioselectivity of palladium-catalyzed hydrostannation reactions involving D-glucal derivatives is influenced by modifications to the olefin moiety. [] Specifically, the presence of a neighboring acetyl group can induce chelation with the palladium catalyst, directing the stannyl group to add at a specific position. [] Additionally, the electronic nature of substituents on the alkene can also impact the regioselectivity of the reaction. [] This highlights the potential for fine-tuning the regiochemical outcome of D-glucal hydrostannation by modifying the olefin substituents.
Q12: Are polymers incorporating D-glucal units susceptible to degradation?
A13: Yes, polymers containing D-glucal units can exhibit susceptibility to degradation. For instance, copolymers of methyl methacrylate (MMA) and a D-glucal-derived cyclic ketene acetal (CKA) showed a tendency towards hydrolytic degradation. [] This susceptibility arises from the presence of ester linkages within the copolymer structure, originating from the ring-opening polymerization of the CKA monomer. []
Q13: Do copolymers of tri-O-acetyl-D-glucal and itaconic anhydride exhibit antimicrobial activity?
A14: Yes, research indicates that copolymers synthesized from tri-O-acetyl-D-glucal and itaconic anhydride demonstrate promising antimicrobial activity. [] The minimum microbicidal concentration-broth dilution method was used to evaluate the antimicrobial efficacy of these copolymers. [] The study found that both the homopolymers and copolymers of these monomers exhibited excellent antimicrobial properties. [] This discovery highlights the potential of these copolymers as novel antimicrobial agents.
Q14: What analytical techniques are used to characterize the products of D-glucal reactions?
A14: Various analytical techniques are employed to characterize the products of D-glucal reactions. These include:
- Nuclear Magnetic Resonance (NMR) Spectroscopy: Utilized to determine the structure, stereochemistry, and purity of D-glucal derivatives. [, , , , ]
- Mass Spectrometry (MS): Employed to confirm molecular weights and analyze fragmentation patterns of D-glucal-derived products. []
- X-ray Crystallography: Used to elucidate the three-dimensional structures of crystalline D-glucal derivatives, providing detailed information about bond lengths, angles, and conformations. [, ]
Q15: What is the potential for biodegradability of polymers incorporating D-glucal units?
A16: Polymers incorporating D-glucal units, particularly those with ester linkages, show promise for biodegradability. [] This characteristic stems from the inherent susceptibility of ester bonds to hydrolysis, making them amenable to breakdown in biological environments. []
Disclaimer and Information on In-Vitro Research Products
Please be aware that all articles and product information presented on BenchChem are intended solely for informational purposes. The products available for purchase on BenchChem are specifically designed for in-vitro studies, which are conducted outside of living organisms. In-vitro studies, derived from the Latin term "in glass," involve experiments performed in controlled laboratory settings using cells or tissues. It is important to note that these products are not categorized as medicines or drugs, and they have not received approval from the FDA for the prevention, treatment, or cure of any medical condition, ailment, or disease. We must emphasize that any form of bodily introduction of these products into humans or animals is strictly prohibited by law. It is essential to adhere to these guidelines to ensure compliance with legal and ethical standards in research and experimentation.